Earth's mantle: what's going on deep beneath our feet?
We know that the ground we walk on is made of solid rock (unless we happen to wander into a patch of quicksand …). But what about the layers of Earth a bit deeper beneath our feet?
Earth’s interior is made of several layers. The surface of the planet, where we live, is called the crust—it’s actually a very thin layer, just 70 kilometres deep at its thickest point. The crust and the lithosphere below (the crust plus the upper mantle) is made of several ‘tectonic plates’. These move slowly across the surface of the planet, and most of Earth’s volcanoes and earthquakes occur at the boundaries between tectonic plates.
Deep in the centre of the planet is the ‘inner core’, which we think is made of solid iron and nickel. This is surrounded by the ‘outer core’, which is also made of iron and nickel, but is molten. Convection currents in the outer core create Earth’s magnetic field.
And between the outer core and the crust is the mantle, which, at around 2,900 kilometres thick, accounts for the bulk (around 84 per cent by volume) of the planet. Carrying Earth’s internal heat to the surface, the convecting mantle creeps like tar on a hot day. This overturning is the ‘engine’ that drives our dynamic Earth—it’s what makes our planet’s geology so interesting, as it enables the movement of tectonic plates. Without it, we wouldn’t have volcanoes, earthquakes … and actually, Earth wouldn’t be able to sustain life.
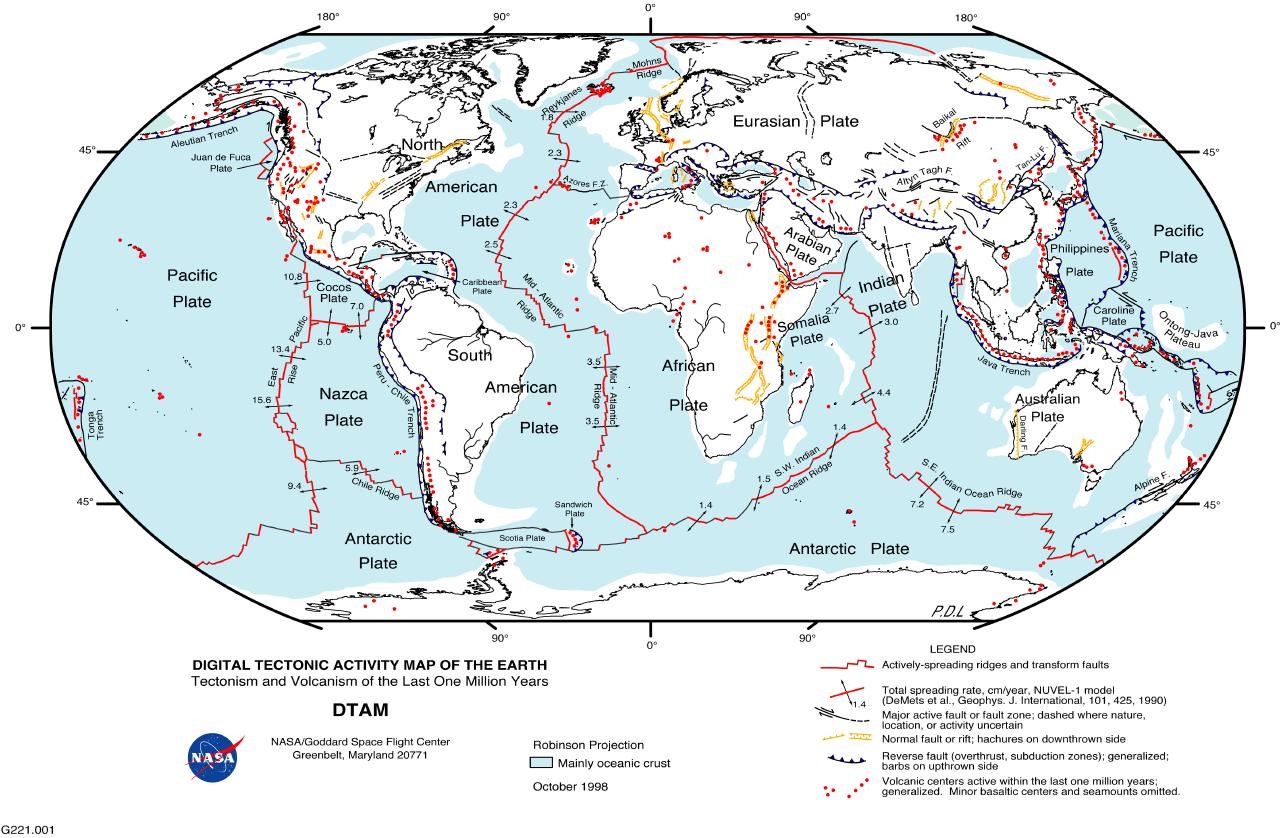
The mysteries of mantle dynamics are what the Australian Academy of Science 2018 Anton Hales Medal winner, Dr Rhodri Davies, spends his time investigating.
He uses advanced computing tools to develop models of mantle dynamics, helping us to understand the mantle’s behaviour and how it influences Earth’s surface. These models combine large-scale geophysical and geochemical datasets with knowledge of how individual minerals behave under certain temperature and pressure conditions to shed light on mantle structure, provide constraints on how the mantle flows, and demonstrate how this flow drives volcanism and other features at the surface.
We know that most of Earth’s volcanoes lie at tectonic plate boundaries, where plates:
- move apart, as is currently occurring between Australia and Antarctica
- move towards each other with one sliding back into the underlying mantle, as at the northern edge of Australia’s tectonic plate beneath Papua New Guinea and Indonesia
- slide past each other, which is occurring at the infamous San Andreas fault in California.
Some volcanoes, however, lie within tectonic plates, far away from these boundary processes. These are called intra-plate volcanoes. Many of these are caused by mantle plumes—regions of hot rock that flow upwards from Earth’s core-mantle boundary towards its surface. In doing so, they carry molten rock material containing a message from Earth’s deep mantle; a message that Dr Davies’ work allows us to decipher. This has helped solidify theories regarding the processes that create intra-plate volcanic island chains.
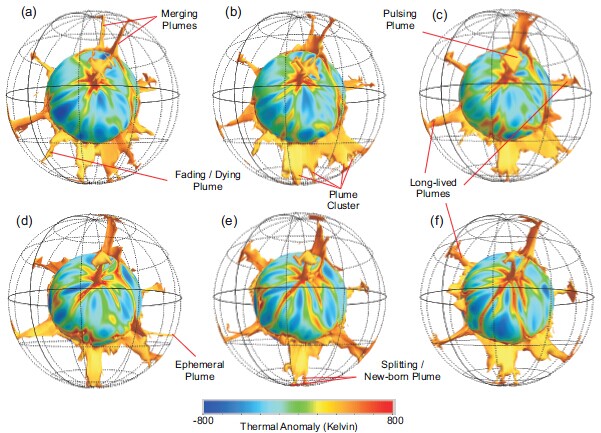
For example, he has combined observations from several fields to show that volcanic chains within Australia formed as the Australian tectonic plate drifted to the north over several mantle plumes. This resulted in a string of volcanoes that traverse the continent from north to south, formed between 34 and 9 million years ago. Believe it or not, the now tectonically sleepy Australian continent houses one of the world’s most extensive intra-plate volcanic regions, with eruptions on the mainland as recently as around 5,000 years ago.
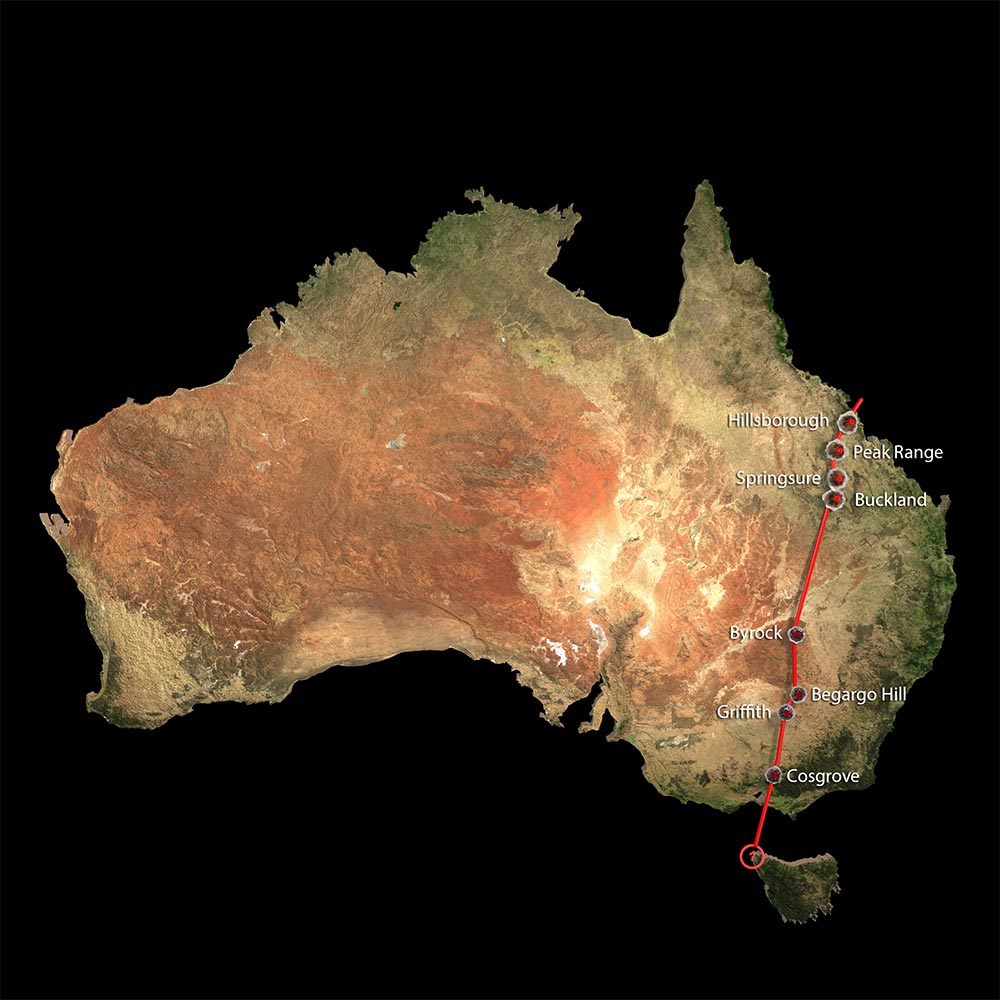
The Hawaiian archipelago is believed to have formed via a similar process. Hawaii sits at the south-eastern limit of a chain of volcanoes and submerged seamounts which get progressively older towards the northwest. This chain splits into two at the island of Oahu and Davies and his group recently found that this split occurred due to a shift in the Pacific Plate’s direction, roughly three million years ago.
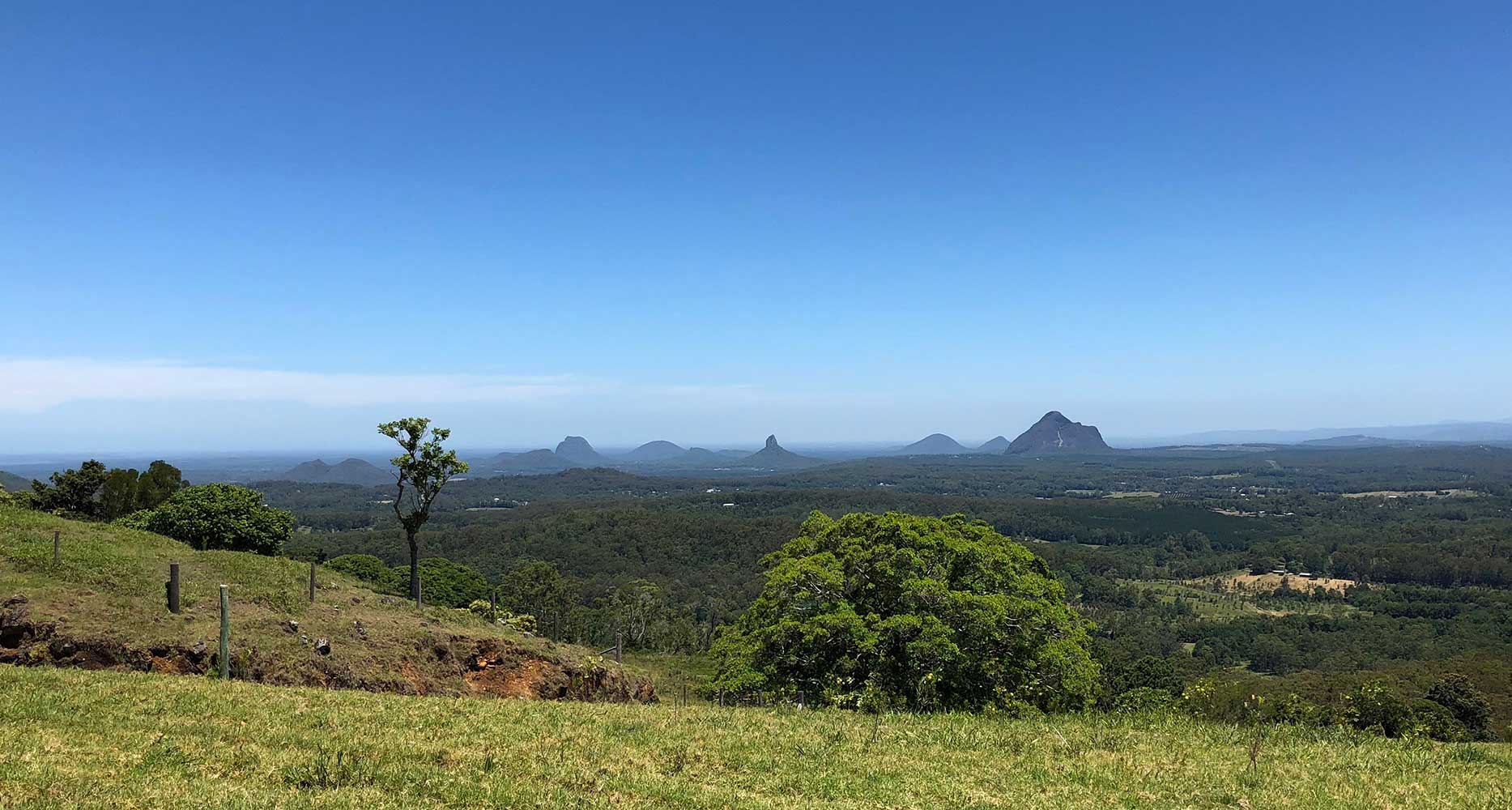
Incorporating all these factors to create models of the way the mantle behaves improves our understanding of the way our planet works. This helps us explain the processes that result in Earth’s unique and spectacular geology and allows us to better understand the planet’s evolution since its formation more than 4.5 billion years ago.