All about DNA
All living things on Earth, in all of its diversity and complexity, are essentially built from the same four building blocks. They’re arranged in a myriad of different ways, of course, but the blueprint for building ‘you’ uses the same components as the blueprint to build a bacterium: we are all built from DNA.
Our DNA (Deoxyribonucleic acid) is found in the nucleus of almost every cell in our body (there are a few exceptions, such as red blood cells, which don’t have a nucleus). DNA is a long molecule, made up of lots of smaller units. To make a DNA molecule you need:
- nitrogenous bases—there are four of these: adenine (A), thymine (T), cytosine (C), guanine (G)
- carbon sugar molecules
- phosphate molecules
If you take one of the four nitrogenous bases and put it together with a sugar molecule and a phosphate molecule, you get a nucleotide base. The sugar and phosphate molecules connect the nucleotide bases together to form a single strand of DNA.
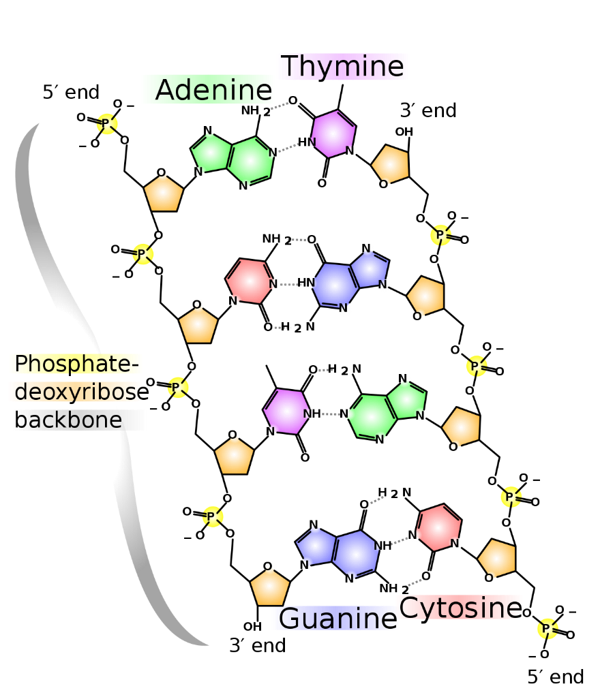
Two of these strands then wind around each other, making the twisted ladder shape of the DNA double helix. The nucleotide bases pair up to make rungs of the ladder, while the sugar and phosphate molecules make the sides. The bases have different shapes and pair up together in specific combinations: A pairs with T, and C pairs with G to make base pairs.
Put three billion of these base pairs together in the right order, and you have a complete set of human DNA—the human genome. This amounts to a DNA molecule about a metre long. You have two copies of the genome, one from your father and one from your mother. The metre-long sequence is cut up into 23 bits, which are then tightly packaged as chromosomes in each of our microscopic cells.
The DNA base pair sequence of all people is nearly identical—that’s what makes us all humans. However, there are small differences in the order of the six billion base pairs in everyone’s DNA that cause the variations we see in hair colour, eye colour, nose shape and so on. No two people have exactly the same DNA sequence. Identical twins are a bit of an exception to this rule, because they came from a single egg that split into two, forming two copies of the same DNA. However, while these DNA sequences may be identical, modifications to how the DNA sequence is ‘read’ can change how genes are expressed between identical twins, especially as they grow older.
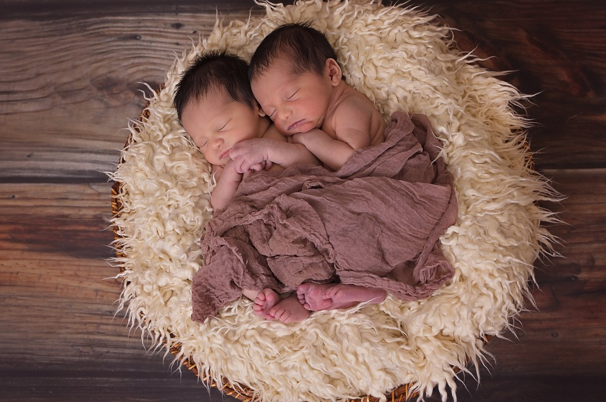
Three billion: why so many?
Three billion is a lot of base pairs. They aren’t just random lists of information though. Rather, within this long string, there are distinct sections of DNA that affect a particular characteristic or condition. These stretches of DNA are known as genes. Their base pair sequence provides the instructions for bringing together the right amino acids in the right order to build a protein. Some genes are small, only around 300 base pairs; others contain over one million.
Genes make up only around 1.5 per cent of our DNA—the rest is extra that initially didn’t appear to have any specific purpose and was dubbed ‘junk DNA’. Turns out, though, that at least some of this ‘junk’ is actually pretty useful—it’s used to define where some genes start and finish and to regulate how the genes behave. While most of the junk DNA comes from copies of virus genomes that invaded our distant ancestors, new studies suggest much of this DNA may have also acquired new functions over time.
Genes contain information to make proteins
Within a gene, the base pairs are read in sets of three and those sets are called codons. These are triplets of base pairs that provide a ‘code’ for one of the 20 different amino acids. Based on the sequence of codes, amino acids are joined together into strings called polypeptides, which then arrange themselves or combine together to build proteins.
Proteins (and RNA) build all living structures as well as acting as catalysts (enzymes) that control biochemical reactions. Proteins build tissues, and tissues build the organs that make up our body. The genes that determine brown eye colour, for instance, contain instructions for the cells in the iris of your eye to make proteins that make brown-coloured pigments. A different sequence of bases would spell a different message, making different proteins and resulting in blue eyes—rather like spelling out a different sentence using the same letters of the alphabet.
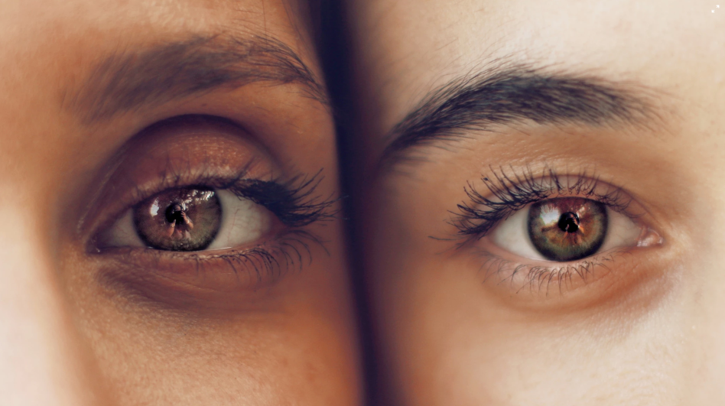
Genes can be switched on or off
If every cell in our body contains the same DNA, how do we end up with the complex arrangement of different cells that is the human (or any other creature’s, for that matter) body?
The secret is that although every cell contains the same sequence of genes, not every gene is ‘switched on’ or expressed in every cell. The cells that make pigment in the eye also contain the genes for making tooth enamel or liver cell proteins, but fortunately don’t do so because those genes are inactive in the eye cells. There are stretches of DNA that do not code for proteins, but rather act as the ‘punctuation’ within the genome, controlling the functioning of genes and other processes.
It’s all of this—the genes plus the ‘punctuation’ plus the ‘junk’—that makes up our genome. In an amazing feat of science, we’ve been able to map out almost the entire human genome (there’s just a few difficult gaps still left to fill), examining which genes are responsible for producing different proteins. Thanks to this map, there’s a whole lot more we can learn about the blueprints of our bodies—and we’re learning more every day.