Understanding gravity—warps and ripples in space and time
Expert reviewers
Essentials
- Isaac Newton described the effects of gravity, but didn’t propose a mechanism for how it worked
- Albert Einstein proposed that massive objects warp and curve the universe, resulting in other objects moving on or orbiting along those curves—and that this is what we experience as gravity
- This theory, general relativity, has led to a number of predictions that have held up to experimental testing
- One prediction of this theory is that ‘gravitational waves’ ripple through the universe, but Einstein thought they would be too small to detect
- In February 2016 the direct measurement of gravitational waves was announced. This provides us with a new method for exploring the universe
- In its current form, general relativity is incompatible with quantum mechanics—signalling that a shift in our understanding may be on the horizon
Take a moment to observe the effects of gravity. Lift your arm and feel how you are compelled to drop it again. Gravity is always there—it’s stable, it’s permanent, it’s unchanging. Or is it?
For hundreds of years we’ve been able to predict the effects of gravity. But we had no idea how it worked until Einstein stepped in, painting a strange and unintuitive picture. In Einstein’s view, gravity is far from a static, unchanging force—it is a fundamental part of the structure of the universe, which curves and twists and ripples as objects move and rotate and jostle about.
The predictions of Einstein’s theories have been validated time and time again. And now, 100 years after the formulation of his theory of gravity, another one of its predictions—gravitational waves—has been directly measured, despite Einstein’s belief that we’d never be able to do this.
In this topic we’ll explore Einstein’s dynamic vision of gravity, including the recently measured phenomenon of gravitational waves. If you’re unfamiliar with relativity GLOSSARY relativityThe general idea that the results of experiments do not depend on the states of motion of observers , some of these concepts may boggle your mind. If so, we encourage you to keep pushing onwards, as it’s one of the greatest journeys in the history of science.
Let’s begin by looking at why Newton’s laws didn’t provide a complete picture of gravity.
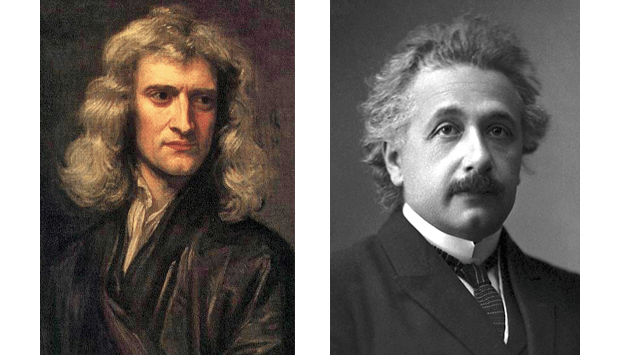
Newton and the laws of gravity
Newton published one of the most celebrated works of science, the Principia, in 1687. In it, he described that the force that pulls objects towards the ground is the very same force that underlies the motion of the planets and stars.
To come to this conclusion, Newton imagined taking an object far from the surface of Earth, and throwing it. If you throw it with too little momentum, it will fall towards Earth, captured by gravity like we are ourselves. If you throw it with too much momentum, it will speed away from the planet, beginning its journey into the reaches of space. But with exactly the right momentum, you can throw it so that it falls continuously around Earth, around and around in an eternal tug-of-war. The object tries to continue in the path you threw it, but gravity keeps on pulling it back in. With the right balance, the object is now in orbit around Earth—just like the moon, or like Earth around the sun.
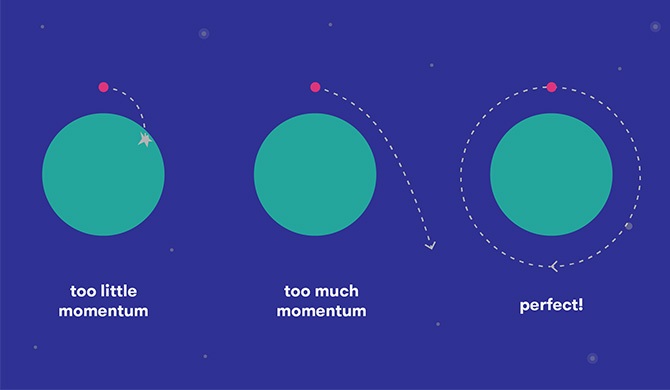
Newton formulated this insight into a mathematical equation, known today as the law of universal gravitation. When combined with knowledge of geometry and Newton’s other equations of motion, we can use it to make predictions about the movement of the planets, or the paths of comets, or how much force is needed to get a rocket to the moon.
We acknowledge Newton not just because of his idea, but because he formulated that idea into an equation that made predictions with greater accuracy than ever before. But it wasn’t perfect—Newton’s equations produced some incorrect predictions, and, more importantly, he didn’t describe how gravity works the way it does. Newton was well aware of this when he said,
Gravity must be caused by an agent acting constantly according to certain laws; but whether this agent be material or immaterial, I have left to the consideration of my readers.Isaac Newton
Distortions in space and time
More than 200 years after the Principia was published, the world was still without an understanding of gravity’s mechanism. Enter Albert Einstein—a man who was to change the world in so many ways. But before we get to his work, we’ll have to take one more detour.
You can’t tell if you’re moving (at a constant rate)
In 1632, even before Newton published his now-famous work, Galileo Galilei wrote about the relative motion of objects familiar in his time: ships.
If you are in a closed room on a ship sailing at a constant speed and the ride is perfectly smooth, objects behave as they would on land. There’s no physical experiment you could conduct to tell whether you’re moving or stationary (assuming you’re not peeking out of a porthole). This is the core idea behind relativity, and is the same reason why we don’t feel our planet’s movement around the sun, or our solar system’s movement through the galaxy.
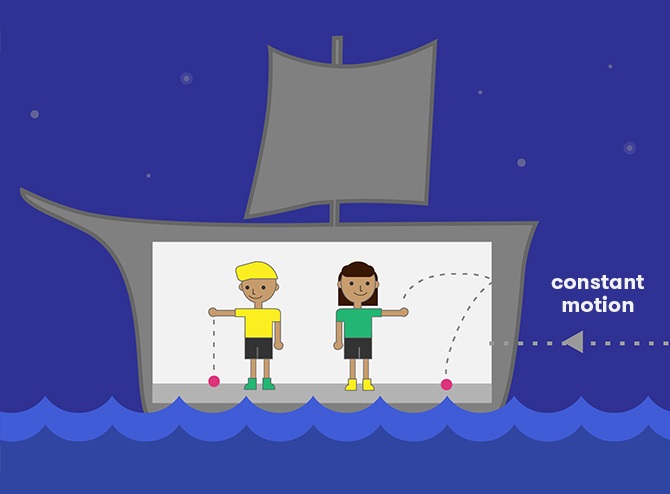
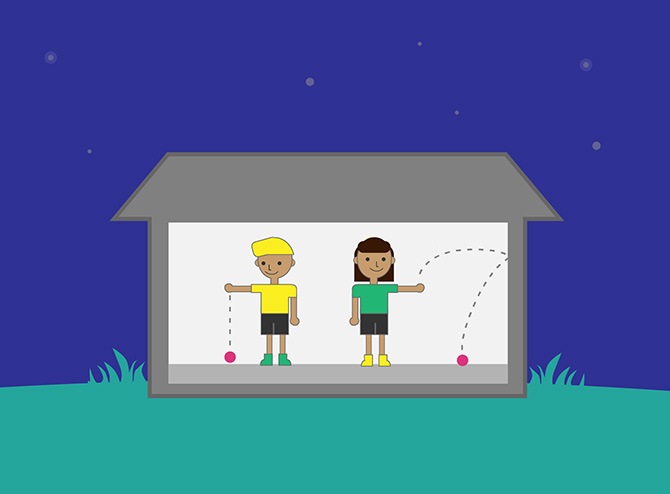
Space and time are linked
Almost 300 years after Galileo, Einstein pondered the consequences of relativity in the context of an important factor: the speed of light. He wasn’t the only person who was pondering these topics—other physicists at the time were aware that there were unanswered questions on this front. But it was Einstein who formulated a theory—his theory of special relativity GLOSSARY special relativityEinstein’s theory regarding the relationship between space and time, the constancy of the speed of light, and the fact that physics must be the same in all uniform states of motion —to explain existing phenomena and create new predictions. At first, special relativity may not seem to have much to do with gravity, but it was an essential stepping stone for Einstein for understanding gravity.
Moving clocks tick more slowly
Experiments during Einstein’s time had shown that the speed of light appeared to be constant. No matter how fast you try and catch up, light always appears to zip away from you at almost 300,000,000 metres per second.
Why is this important? Well, let’s imagine constructing a clock out of light itself. Two mirrors are placed opposite each other, and a “tick” of the clock is the time it takes for a particle of light to travel from one side to the other and back.
Now let’s imagine that your friend, who’s on a spaceship zipping past Earth, has one of these clocks. For your friend, the clock seems to be working normally—the particles of light travel up and down, as expected, and time proceeds in its usual fashion. But from your perspective, watching the ship pass by, the light is moving both up and down and to the side, with the ship. The light travels a longer distance with each tick.
Stationary vs moving light clocks
(in slow motion)
As seen from inside the spaceship
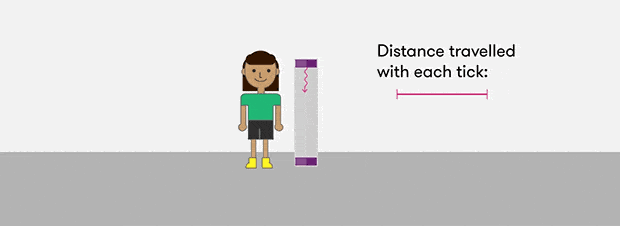
As seen by a stationary observer
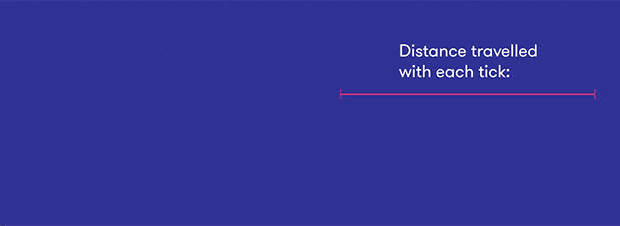
So if, for the space traveller, light travels at 300,000,000 m/s but only has to travel up and down; and to the Earthbound observer, light travels at 300,000,000 m/s, but must travel a longer, diagonal distance; then for the Earthbound observer, the clock takes longer to “tick”.
This effect is called time dilation GLOSSARY time dilationThe slowing down of time for one observer relative to another . The faster you travel through space, the slower you travel through time.
Perspective matters
But whose time is really slowed down? Is it the person on Earth, watching his friend zip past in her spaceship? Or the astronaut, who argues she’s staying still while the Earth flies by?
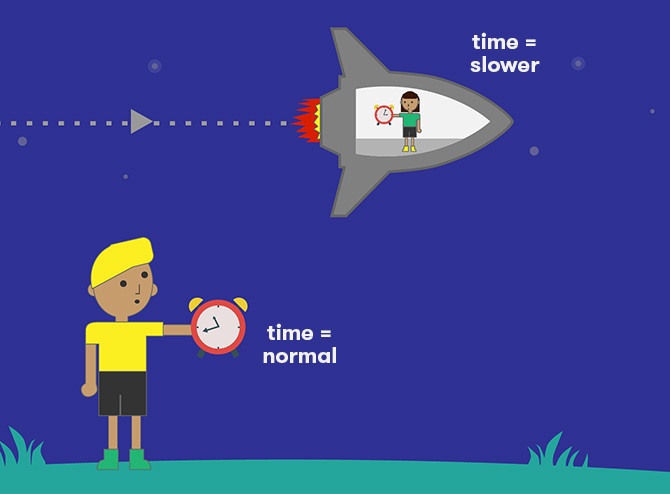
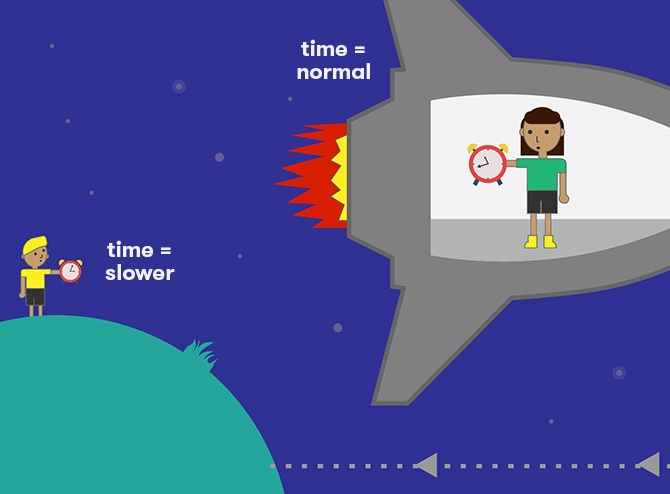
Strangely enough, both viewpoints are valid—but only while both are in constant motion.
To illustrate, let’s assume that when the astronaut left Earth, she and her friend were the same age. When she leaves, the spaceship accelerates away from Earth. When she returns, the spaceship decelerates to avoid a crash landing. In both leaving and returning, the spaceship changes its frame of reference GLOSSARY frame of referenceThe physical environment of an observer that involves their state of motion. A person travelling in one car is in a different frame of reference than someone travelling in a car going a different speed or direction, or a pedestrian at the side of the road, or someone travelling overhead in a plane, etc. , and our astronaut can feel the change of motion. Experiments conducted inside the spaceship during acceleration and deceleration would show that something’s changing. This breaks the symmetry of the situation, and when the spaceship lands back on Earth, our astronaut really will be younger than her Earthbound counterpart.
The effects are only noticeable if they were travelling really, really fast—but it’s still true to say that when today’s astronauts and fighter pilots return from a high-speed mission, they will have aged a teeny-tiny bit less than the rest of us did during that mission.
The four dimensions of spacetime
Following from this, rather than thinking of three dimensions of space and one separate dimension of time, we can consider them as four dimensions of “spacetime”. The faster you travel through space, the slower you travel through time, and vice versa.
Moving objects contract in space
Another consequence of special relativity is that fast-moving objects appear to contract in size, in the direction of their motion. (And again, this gets flipped around depending on whose perspective you’re looking from.)
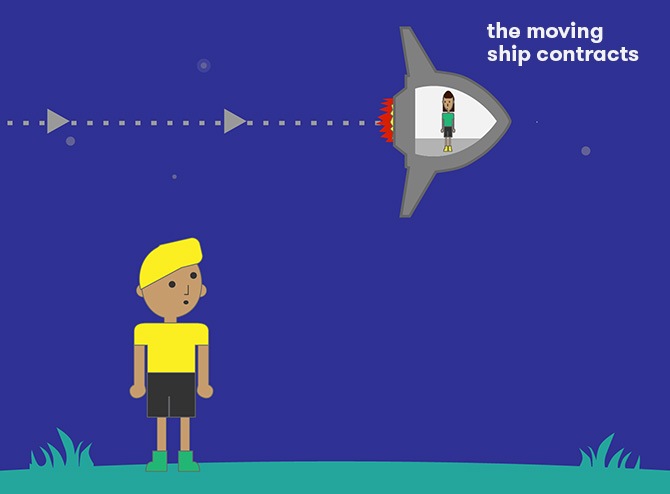
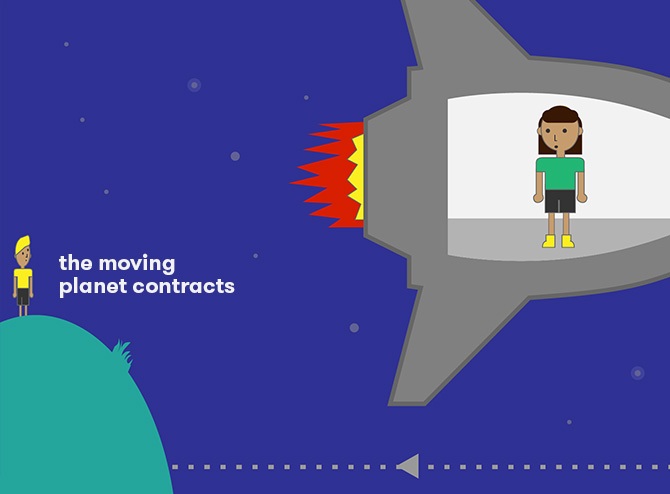
This follows from the distortion of time—after all, you can measure the length of something by the amount of space something travels through time (e.g. light-years, light-seconds). And while it’s tricky to imagine measuring the length of a moving object from someone else’s perspective, length contraction is a real, physical effect, and not just an outcome of imprecise measurements.
Unlike the age differences that can arise from time dilation, there are no residual effects due to length contraction once the moving object and the observer are reunited.
Understanding gravity
Einstein’s description of gravity leads to situations just as bizarre as special relativity—time travel included!
Acceleration and gravity can be indistinguishable
Imagine waking up in a spaceship, accelerating through space. Just as you’re pushed back in the seat of an accelerating car, the accelerating spaceship pushes you to the side opposite the one it’s accelerating towards. At a certain rate of acceleration, a set of scales could tell you that you weigh exactly the same as you do when you’re at home on Earth.
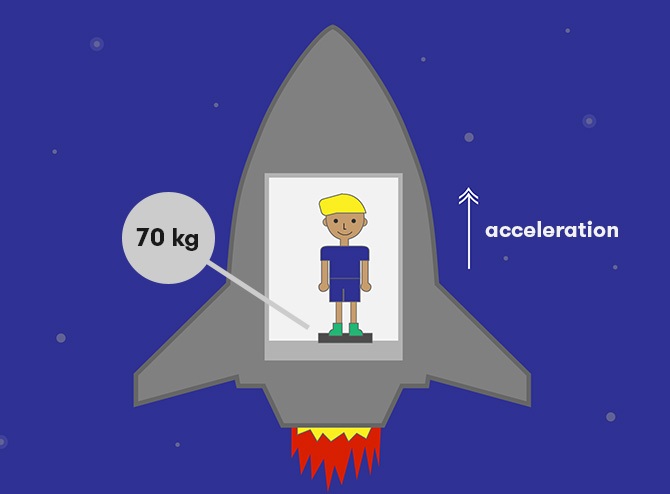
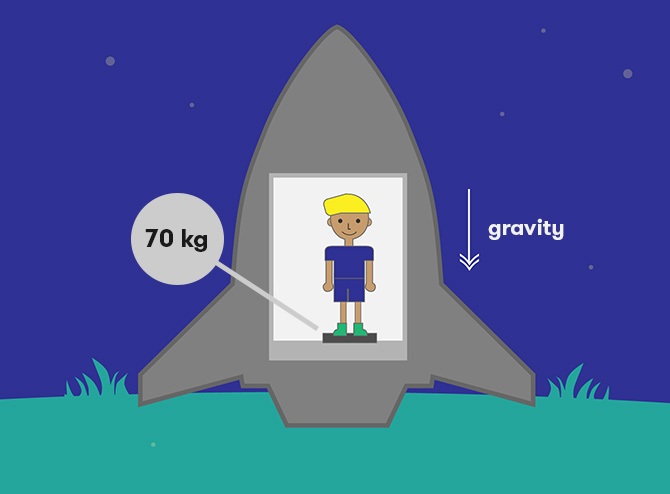
Is there any physical experiment you could do within the confines of your spaceship to tell whether you really were accelerating through space (assuming there were no windows to look out from), or if, instead, you were inside a spaceship stationary on the surface of Earth? Einstein said no—just as Galileo imagined the indistinguishability between a person inside a smooth-sailing ship (confined without windows) and a person on land, Einstein realised that the effects of acceleration and gravity were indistinguishable too. This is called the equivalence principle GLOSSARY equivalence principleThe effects of being in a gravitational field are indistinguishable from the effects of being in an accelerated frame of reference .
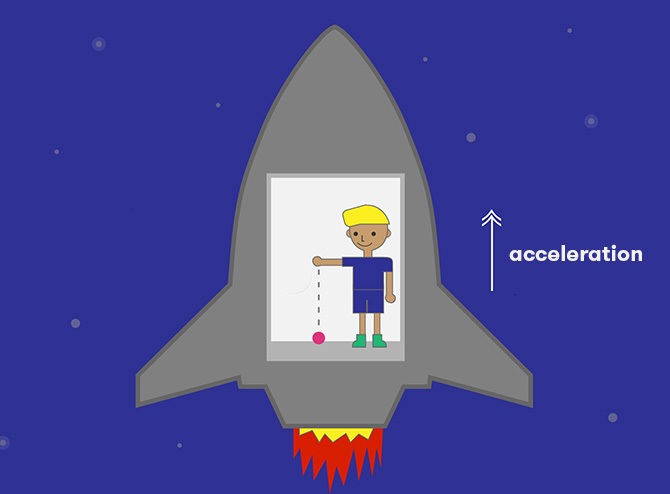
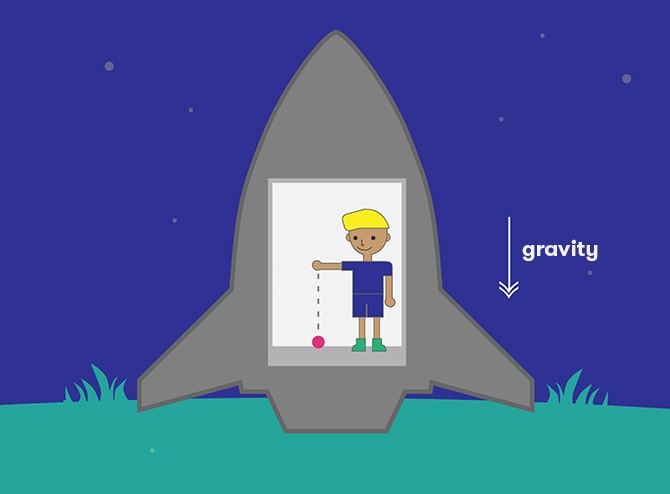
Space warps under accelerated motion
Once Einstein had formulated the equivalence principle, gravity became less mysterious. He could apply his knowledge of acceleration to better understand gravity.
You may know that acceleration doesn’t always mean a change in speed, like when you speed up in a car, pushing you to the back of your seat. It can also mean a change in direction, like when you go round a roundabout, causing you to lean towards the side of the car.
To extend this further, let’s imagine a cylindrical carnival ride where you and your fellow passengers are pinned to the outer surface. The cylinder is rotated faster and faster until the acceleration eases and the movement stays constant. But even once the speed is constant, you still feel the accelerated motion—you feel yourself being pinned to the outer edge of the ride.
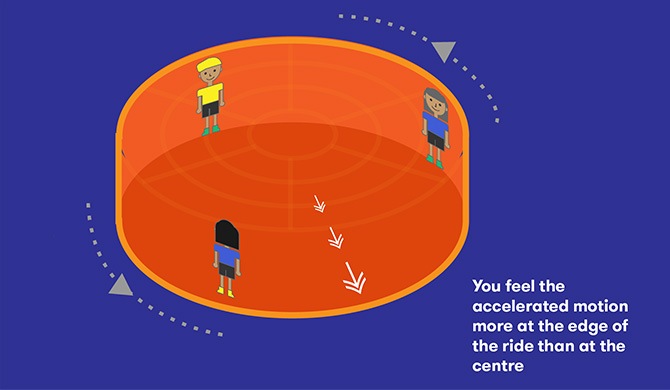
If this spinning ride was large enough and moving at a fast enough rate, you’d start to notice some bizarre effects inside the ride itself, not just from the point of view of someone standing outside it.
With every rotation, those at the edge of the ride travel the full circumference of the cylinder—while at the very centre, there’s hardly any movement at all. So if someone stood in the very centre of the ride (perhaps held by a brace, stopping them from falling to the edge), they would notice all those weird effects we saw under special relativity—that those on the edge will contract in length, and their clocks will tick at a slower rate.
Gravity is the curvature of spacetime
The equivalence principle tells us that the effects of gravity and acceleration are indistinguishable. In thinking about the example of the cylindrical ride, we see that accelerated motion can warp space and time. It is here that Einstein connected the dots to suggest that gravity is the warping of space and time. Gravity is the curvature of the universe, caused by massive bodies, which determines the path that objects travel. That curvature is dynamical, moving as those objects move.
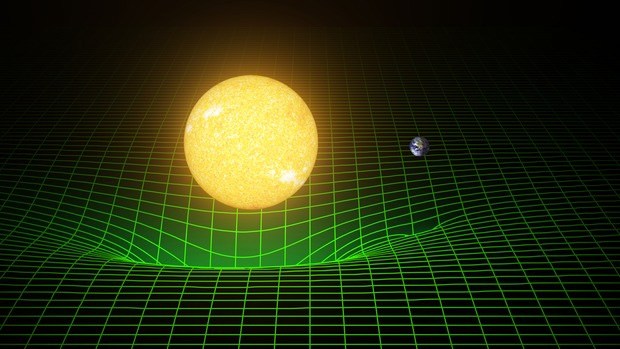
This theory, general relativity GLOSSARY general relativityEinstein’s theory of gravity , predicts everything from the orbits of stars to the collision of asteroids to apples falling from a branch to the earth—everything we have come to expect from a theory of gravity.
Spacetime grips mass, telling it how to move... Mass grips spacetime, telling it how to curvePhysicist John Wheeler
The success of general relativity
Just as Newton’s formulation of the laws of gravity were valuable because of their predictive power, the same goes for those of Einstein. To date, his predictions—as strange as they may sound—have all stood the test of time.
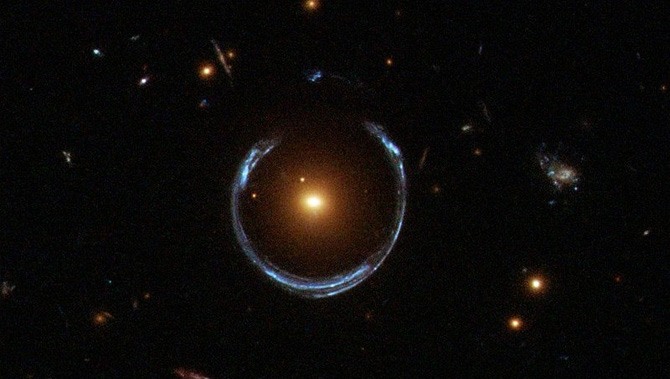
Gravitational waves
Echoes of cataclysm from far away
Imagine two very massive objects, such as black holes. If those objects were to collide, they could potentially create an extreme disturbance in the fabric of spacetime, moving outwards like the ripples in a pond. But how far away could such waves be felt? Einstein predicted that gravitational waves existed, but believed they would be too small to detect by the time they reached us here on Earth.
So it was with great excitement that on February 11 2016, the scientific community was abuzz with the announcement that a gravitational wave GLOSSARY gravitational waveRipples in spacetime that propagate outwards like waves had been detected. We needed instruments capable of detecting a signal one-ten-thousandth the diameter of a proton (10-19 meter). That’s exactly what the Laser Interferometer Gravitational-Wave Observatory (LIGO) equipment, operated by the California Institute of Technology and the Massachusetts Institute of Technology, can do.
The LIGO experiment
In the LIGO experiment, a laser is directed into a large tunnel structure. The laser beam is split so that half of it travels down one of the 4-kilometre-long ‘arms’, and the other half travels down the other 4-kilometre arm at the exact same time. At the end of each arm, a mirror reflects the light from the laser back to where it came from, and the two beams merge back into one.
Normally, the laser beams should recombine at exactly the same time. But if a gravitational wave comes rippling through space while the detectors are switched on, that ripple will stretch one arm of the L-shaped structure before stretching the other. The gravitational wave distorts the passage of the light, resulting in a particular kind of interference light pattern detected at the end.
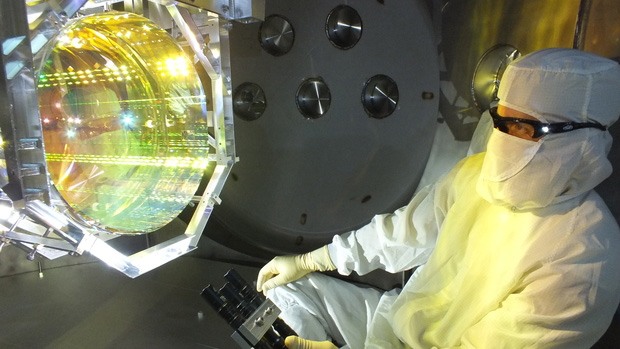
On 11 February 2016, the LIGO teams announced the direct discovery of a gravitational wave matching the signal predicted from the collision of two black holes.
Gravitational wave astronomy
The successful LIGO experiment has ushered in a new era of astronomy. Before now, astronomers have largely focused on the study of the electromagnetic spectrum (including light and radio waves). We’ve been able to discover a huge amount about our universe through that work, but now we have a brand new way to study the universe.
The discovery of gravitational waves gives astronomers a new ‘sense’ with which to explore the universe, and so there will almost certainly be surprises ahead. What we do know is that this technique will allow us to better understand the most massive objects in the universe such as black holes, neutron stars, and supernovae; and it will provide us with a new window to study how the universe formed.
Is our understanding complete?
While Einstein’s theory of gravity has been validated by experiment after experiment, this does not mean our understanding is complete. In fact, we know that something’s not quite right.
One unanswered question is whether or not gravity is propagated by the graviton—the proposed (but so-far undetected) particle responsible for gravitational interactions. Even more pressing, we know that general relativity is, in its current form, incompatible with the other pillar of modern physics: quantum mechanics GLOSSARY quantum mechanicsA branch of physics that explains how the universe works on incredibly tiny scales (atomic and subatomic) . This is an indication that one or both theories are incomplete, or that we’re missing some other key component.
Whether or not Einstein’s theory of gravity will remain unchanged is not known. But it has produced many unexpected, unintuitive predictions that have been confirmed again and again for over a hundred years. That’s the sign of a great scientific theory—it makes predictions that may not be able to be proven at the time, but stand up to rigorous testing. This has been one of the greatest journeys in the history of science, involving not just Newton and Einstein, but thinkers and doers all around the world who have worked to put these theories to the test.
Even so, the schism between relativity and quantum mechanics remains. As for what’s next, no one knows with certainty. However, there are a few theories—stringy, loopy, multi-dimensional theories—unproven but with promise of becoming the next milestone in understanding our cosmos.