Journey to the centre of the planets
How much do you know about the planets of our solar system? Perhaps you can recite their order from the Sun, or their sizes from big to small? But does your knowledge go any deeper … literally?
Researchers are attempting to understand planets more intimately by studying their varied and sometimes dangerous interiors. This information can help us understand how different planets formed, why they behave like they do and how they may continue to change.
But hang on, you might say, we haven’t even landed humans on Mars yet, let alone dived under the clouds of the gas giants or cracked through the ice of the dwarf planets—so how can we possibly know what lies beneath?
To find these answers we have to rely on indirect methods. These require the merging of keenly observed astronomy, complex experiments in the lab, theoretical calculations and intelligent guesswork.
Thanks to modern technology—impressive telescopes, satellites and robotics—we can actually gather a lot of information about far-flung exoplanets from here on Earth. By observing their gravity, mass, magnetic fields, surface chemistry and topography, researchers can begin to piece together what is (or may be) happening under the surface.
A planet’s magnetic field protects it from the charged particles (from the sun and elsewhere) that fly through the universe. Earth has a strong magnetic field which most scientists think is due to its hot iron core. As the core rotates it works like a hot dynamo to produce our magnetic field. Mars, however, only has a very weak magnetic field. This would suggest that it does not have a hot iron core, or that it did, but due to Mars’ smaller size, it cooled down more quickly.
Similarly, NASA’s Juno probe, which reached Jupiter in 2016, is revealing the planet’s magnetic field. Data shows that the magnetic field is ‘lumpy’, that is, stronger in some places and weaker in others. Jack Connerney, Juno’s deputy principal investigator, says ‘this uneven distribution suggests that the field might be generated by dynamo action closer to the surface, above the potential layer of metallic hydrogen. Every flyby we execute gets us closer to determining where and how Jupiter’s dynamo works.’
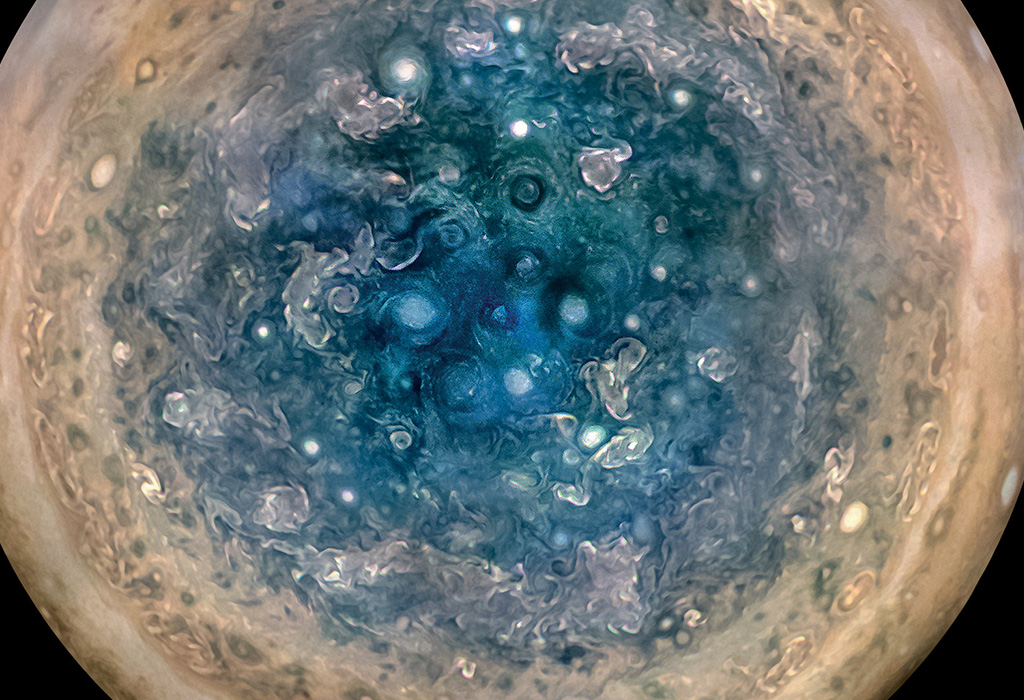
The topography of a planet can also be useful to deconstruct what’s happening underneath. Active volcanoes on a planet’s surface, such as on Earth, indicate a mantle of magma flowing underneath a relatively thin crust. Evidence of extinct volcanoes, like those on Mars, suggests there was a flowing mantle in the past but it’s no longer present. Similarly, the surface of Venus is covered with more than 100,000 seemingly extinct volcanoes. But it wasn’t until 2015 that scientists—studying data collected by the Venus Monitoring Camera back in 2008—were able to establish a significant heat signal, indicating the planet is still active and changing. And while volcanism on Earth mostly marks the boundaries and movements of our planet’s tectonic plates, there are no such patterns observed on Venus.
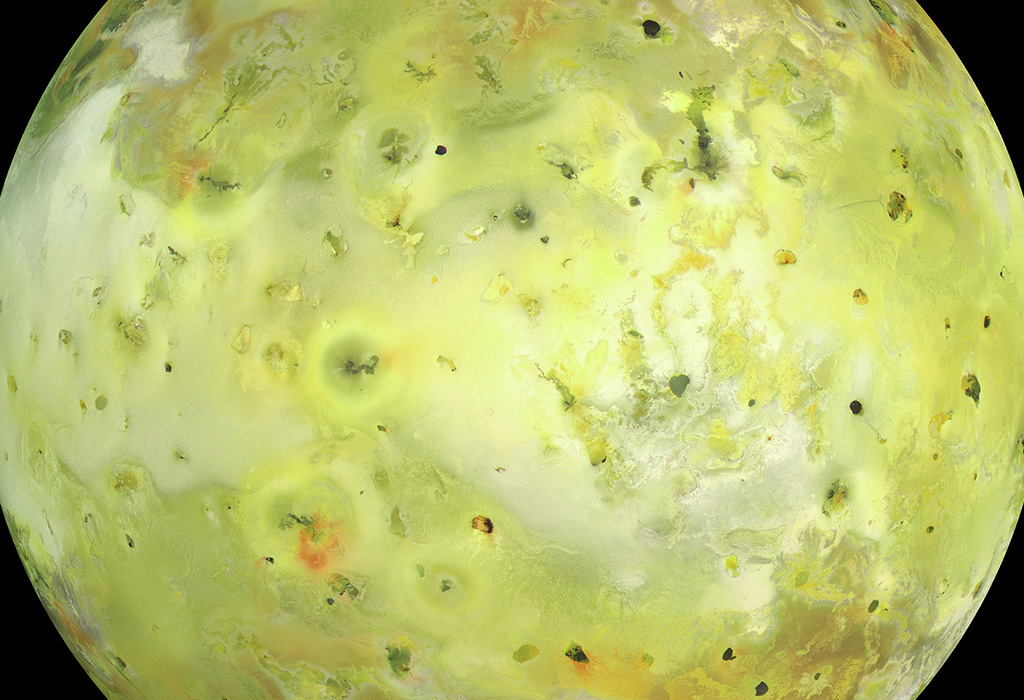
The frequency, duration and size of quakes (earthquakes, moonquakes, marsquakes etc) are also indicators. The more quakes, the more active the interior of the planet. NASA’s InSight Mission to Mars to study marsquakes and meteorite impact activity is expected to launch in 2018. A heat probe will also be placed into the rock to determine the temperature below the surface, which will enable researchers to measure crust thickness and heat-flow. This information should provide a wealth of under-surface information, including the types of rocks and other materials that make up the red planet.
Every planet has its own unique gravity due to its size and mass distribution. Earth, for example, is a rocky planet. In contrast, the gas planets, including Jupiter and Saturn, have deep gaseous atmospheres surrounding highly condensed cores.
The orbit of a spacecraft travelling around a planet is affected by that planet’s gravity. By analysing the small fluctuations in the data of orbiting spacecraft we can more accurately map the gravity field of a planet and also help figure out the planet’s density distribution. NASA’s Juno probe has been orbiting Jupiter since 2016 to understand the origin and evolution of Jupiter, look for a solid planetary core, map magnetic fields, measure water and ammonia in deep atmosphere and observe auroras’. Juno has already discovered that there’s not as much mass concentrated in Jupiter’s centre as first thought and instead its core is more ‘fuzzy’.
The Cassini spacecraft, busy orbiting Saturn since 2004, altered its orbit so that it passed between the planet’s outer rings before plunging into the planet and burning up in 2017. Findings from these two missions is building our knowledge of these planets and what’s happening within them.
Surface chemistry maps of planets can also add more pieces to the puzzle. Surface maps taken of Mercury by the MESSENGER spacecraft revealed remarkable chemical variability, including the existence of distinct geochemical regions that have compositions very different to their surroundings. Larry Nittler, Deputy Principal Investigator of the mission, says these observations ‘will provide critical constraints on future efforts to model and understand Mercury’s bulk composition and the ancient geological processes that shaped the planet’s mantle and crust.’ The surface colour of planetary bodies can also be an indicator of the elements present. The distinct red colour of Mars is due to iron oxide dust, while Neptune looks blue because of methane in its atmosphere.
Once data has been collected, scientists turn their gaze back to the labs. Recent technological breakthroughs—such as high-powered lasers, cutting-edge X-ray sources and high-performance supercomputers—allow researchers to create extreme states of matter, analyse states at the atomic level and run complex simulations.
Three recent studies by researchers at the US Department of Energy’s SLAC National Accelerator Laboratory used these approaches to discover new information about meteor impacts, the cores of giant planets and cosmic particle accelerators. In particular they were able to discover new information about Warm Dense Matter (WDM) —the stuff believed to make up the cores of the giant planets such as Jupiter—including how it forms and why. This includes the fact that methane in the atmosphere of Uranus and Neptune could, when sucked deeper into their interiors, dissociate into its carbon and hydrogen components. This could mean that these giant blue planets have diamond cores.
Some planets—such as our gas giants including Uranus—have conditions so extreme they cannot be recreated in the lab. When this occurs, researchers have to rely on theoretical experiments. This method discovered the phase of ‘super-ionic’ water on Uranus—where the hydrogen ions are separated from the oxygen in water, with the result that charged particles can whiz about. It’s thought that these generate the unusual magnetic field of the planet.
All of the information collected so far is based on what we know about planets in our own solar system. With the Kepler spacecraft continually finding more exoplanets and solar systems orbiting other stars, there is no shortage of new planetary interiors to explore. Who knows what we’ll find next?