Seeing the future: the bionic eye
Expert reviewers
Essentials
- There are several different types of visual prostheses—also known as bionic eyes—being developed around the world
- The technology may restore partial vision to users, but cannot yet restore full vision
- Most current models of bionic eyes encompass both internal implants and external cameras
- Development of bionic eyes involves experts across a wide range of fields (electrical and biomedical engineers, ophthalmologists, vision scientists, clinicians and surgeons, as well as physicists, physiologists, materials engineers and computer scientists)
- The improving technology of bionic eyes could, in the future, potentially address most forms of vision loss
Those of us with healthy vision often take our sight for granted. From admiring masterpieces at the art gallery to being able to find the vegemite in the fridge, we rarely consider how different our lives would be if our vision was impaired. But reduced sight is a reality for more than half a million Australians, who currently experience blindness or low vision in a manner that affects their daily functioning.
The development of a bionic eye is therefore exciting technology. As with other medical bionic devices, however, it is not suitable for everyone. At least, not yet.
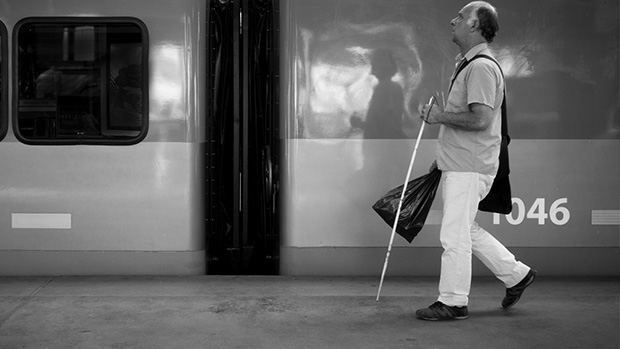
How does a healthy eye work?
Before we delve in to how a bionic eye might work, it helps to have a basic understanding of how a healthy eye functions.
The human eye is a complex and intricate organ. A dome of clear tissue at the front of the eye, known as the cornea, focusses light as it passes through. Behind the cornea sits the iris (the coloured part of the eye), with the pupil at its centre, which lets light into the eye. Muscles (known as the ciliary muscles) are attached to the iris and can alter the size of the pupil, making it bigger or smaller and therefore regulating how much light gets through. After light has passed through the cornea and the pupil, it passes through the lens of the eye. The lens can change shape to allow us to focus on objects that are near or further away. It is the cornea and the lens together that focus the light onto the back of the eye, known as the retina.
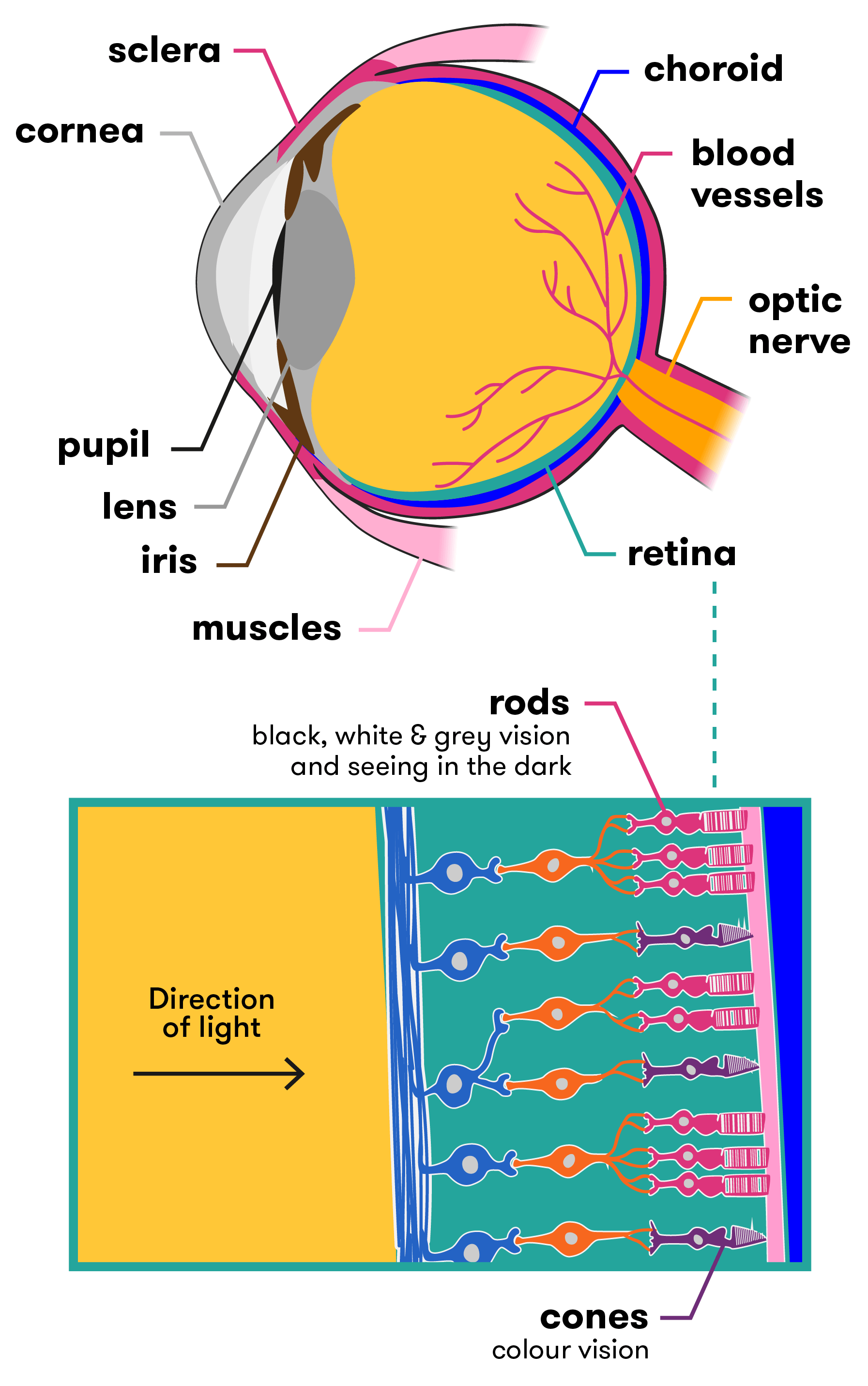
The retina contains millions of light-sensitive cells called photoreceptors. There are two types of photoreceptors—rods (approx. 90 million) and cones (approx. 4.5 million). Rods see in black, white and grey, and assist us to see in the dark. The cones are sensitive to either red, green or blue, and together allow us to see millions of colour variations. Cones need more light to allow to them to work properly. The rods and cones interact with many different types of neurons in the retina to translate what you’re seeing into nerve messages, which are sent to the brain via the optic nerve. The brain then interprets these signals and tells you what you are seeing (car, bike, person etc.).
What is a bionic eye?
The bionic eye is a colloquial name given to a visual prosthesis—an electrical device that assists in restoring a sense of vision to the user. While it’s true that this is futuristic technology, it is important to note the key words here are ‘sense of vision’. This is because the bionic eyes currently under development—of which there are several around the world—cannot restore full 20/20 vision to people, nor give sight to those who have never been able to see before. Most of the bionic eye technologies being developed require a healthy optic nerve and a developed visual cortex—that is, patients need to have been able to see in the past for these devices to work successfully.
There are many different types of vision loss (full/partial/colour) and many causes for this loss (genetics/illness/injury). Some forms of hereditary blindness—such as retinitis pigmentosa—cause loss of vision by destroying the photoreceptors (the rods and cones) but leave most of the other retinal cells intact. Others—such as traumatic eye injury or glaucoma—may result in permanent damage to the optic nerve and visual pathways. It is due to these differences that researchers are working on different types of bionic eye implants, with the two showing the most promise being the retinal (eye-based) implant and the cortical (brain-based) implant.
Retinal-based bionic eyes
Retinal-based bionic eyes are suitable for patients who have lost their vision due to disorders such as retinitis pigmentosa and age-related macular degeneration. Both of these conditions reduce vision due to photoreceptor death, but leave patients with a relatively functional optic nerve (the visual pathway from the retina to the brain), as well as some intact retinal cells. So although patients cannot see, much of the hardware in the eye is still working. Researchers tap in to these functional parts of the eye as part of the bionic technology.
Australia is one of several countries racing to develop this type of bionic eye technology. Researchers across the globe are working on different devices that, while similar in many ways, have key differences in others. Some devices for example are epi-retinal (designed to be placed in front of the retina), others are sub-retinal (for placement behind the retina). The hardware and capacity of each design also varies. Let’s take a look at a few of them.
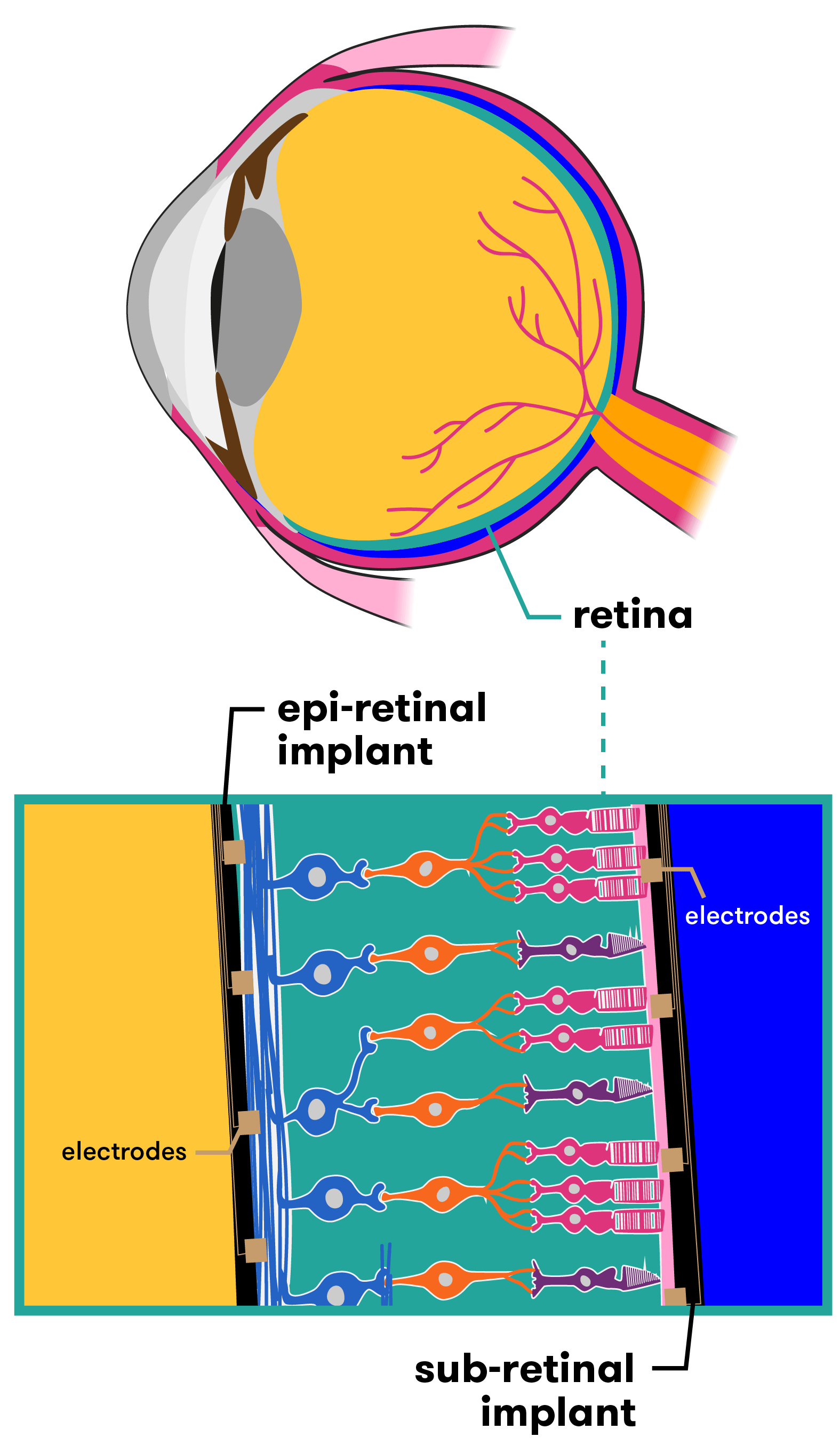
Australia
Bionic Vision Australia (BVA) has been working on several devices to assist people suffering from retinitis pigmentosa and age-related macular degeneration vision loss. The BVA consortium consists of The University of Melbourne, The University of New South Wales, the Bionics Institute, the Centre for Eye Research Australia, NICTA and several other partners. The bionic vision system they have developed consists of both internal and external components.
In its current form, a small camera is attached to a pair of glasses. The camera captures images and sends the data to an externally worn body processing unit. The unit then sends the processed data (via an external wire) to a receiver device implanted in the patient. This receiver passes the information to the retinal implant, which is situated at the back of the eye. Electrodes convert these signals into electrical impulses, stimulating the cells in the retina that connect to the optic nerve. The optic nerve then transmits these impulses through to the vision processing centres of the brain, where they can begin to be interpreted as an image.
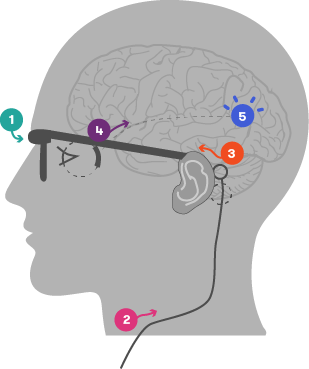
- Camera captures image and transmits data to an external, body worn processing unit
- Data processed and sent to implanted system via external wire
- Implanted receiver passes signals onto retinal implant
- Implanted electrode array stimulates retina
- Electrical signals sent from retina via visual pathway to vision processing centres in the brain
Image courtesy of Bionic Vision Australia (adapted)
In 2012, three people experiencing complete vision loss saw the light—or at least, flashes of it. They were the recipients of BVA’s first bionic eye prototype, a retinal implant with 24 electrodes. Their feedback on what they could see, how the system worked and what could be improved helped researchers refine the technology over the following two years. For example, an external camera was fitted to the implant in 2013, enabling patients to identify basic letters, numbers and shapes. By 2014, the system was more portable, allowing patients to navigate to set points and move around defined obstacles (within a laboratory setting).
The two-year trial proved the safely of the materials, technology and surgical approach. This has paved the way for new technologies currently being developed.
- Wide-View 44 electrode device
- This prototype aims to restore vision to a degree that enables the patient to experience increased mobility and independence. The device will be placed in the suprachoroidal space, which is between the two outer layers of the eye (the choroid and the sclera). This positioning in the outer parts of the eye will protect the retina from potential damage during surgery, while also assisting in maintaining the implant’s position within the eye. A higher-resolution 98 electrode suprachoroidal device is also currently in testing at the University of New South Wales.
- High-Acuity 256 electrode device
- A prototype designed to restore a level of sight that will enable patients to recognise faces and read large print. It will be surgically implanted epiretinally—that is, on the inside surface of the retina. Interestingly, the unit that contains the implant and its associated electrodes is made of inert polycrystalline diamond. Why diamond? Well, trials have shown that diamond with nitrogen added to it is successful in conducting electric currents to stimulate the retina , eliciting natural responses in preclinical studies.
United States of America
American company Second Sight has also developed a retinal implant that provides stimulation to induce visual perception in vision impaired patients. Called the ARGUS II, it works in a similar way to the devices produced by BVA, with a video camera worn on external glasses sending information wirelessly to the retinal implant. These signals are sent to the electrode array, which emits small pulses of electricity. The eye’s damaged photoreceptors are bypassed, while the remaining viable retina cells are directly stimulated. This transmits the information along the still-functioning optic nerve to the brain, which perceives patterns of light. Second Sight has achieved FDA approval for commercialisation in the USA and CE Mark approval in Europe.
By 2016 over 80 vision impaired people in the United States had been fitted with the technology. They were able to see light and dark contrasts, read large print books, and navigate an unfamiliar home, but they were not able to see in colour. So while the device gave them back a ‘sense of vision’, it was not sophisticated enough to lift them out of ‘technical blindness’ (measured at 20/200 – the best users have reported is 20/1000). However, improvements are coming, and the researchers hope the next version of the Argus II will be able to perceive colours, provide clearer images and more fully focus eyesight on a single point (such as a computer screen).
Germany
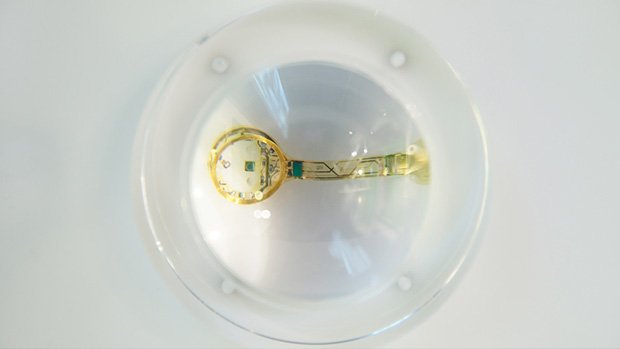
Similarly, researchers in Germany have unveiled their latest addition to the bionic eye ‘family’. The technology was developed by the University of Tubingen in Germany and is known as the Alpha IMS retinal prosthesis.
The Alpha IMS is a retinal implant consisting of a silicon chip approximately 3 x 3mm in size and 70µm thick, which is surgically implanted behind the retina. In this way, it replaces the photoreceptors that have been damaged or lost, and importantly, its sub-retinal location has the potential to exploit the full range of neuronal circuitry in the retina along the way to the optic nerve. This is not yet confirmed however, as the retinal circuitry is reorganised to varying degrees by blindness, so the processing may not be so useful anymore.
This small electrode is wirelessly connected to a tiny computer placed underneath the skin behind the ear. A magnetic coil on the skin allows the implant to be adjusted for brightness, and power is provided via a battery pack.
The Alpha IMS currently has several differences to its competitors. Firstly, it is self-contained, so there is no external camera used. While this results in a less bulky device, there are trade-offs. The advantage of an external camera is the access to large processing power that can be applied to the sign before it is sent to the implant, which can improve the vision experience.
The second difference is that the implant consists of 1500 electrodes (the current ARGUS II only has 60). This has the potential to provide users with extremely high levels of visual acuity and resolution. Interestingly though, testing has shown that despite the larger number of electrodes in the Alpha IMS, the device performed no better in visual tests than the Second Sight Argus II 60 electrode system. The researchers theorised that other factors such as the severity of the retinal degeneration, cortical remodelling, electrode-tissue interface and psychological factors such as a patient’s willingness to participate in intensive rehabilitation may be more important.
It should be noted here that in many ways, discussing and comparing results from the different devices is difficult because of the varying ways that performance is measured by the developers. A consortium, called the Harmonization of Outcomes and Vision Endpoints in Vision Restoration Trials (HOVER) Taskforce, is now trying to develop standardised tests for devices so that results can be more accurately measured and compared.
In clinical trials, eight of the nine patients fitted with the Alpha IMS were able to detect mouth shapes (smiles/frowns), signs on doors, small objects such as phones and cutlery—even if a glass of wine was red or white.
A further potential benefit is that because the Alpha IMS has an in-built sensor that works to directly gather its imagery from the light that passes into the eye (rather than from an external camera), users are able to simply move their eyeballs from side to side to pick up stimuli. This is easier than having to turn their head so that a camera is directly facing the image for capturing.
English woman Rhian Lewis, who suffers from retinitis pigmentosa, received an Alpha IMS retinal implant as part of an ongoing trial at Oxford’s John Radcliffe hospital. During tests, Rhian was able to recognise the time on a large cardboard clock. Describing the experience of being able to see the time she said ‘Honest to God, that felt like Christmas Day.’
France
In France too, the technology is progressing, with the company Pixium Vision working on both epi-retinal (in front of the retina) and sub-retinal (behind the retina) devices.
Direct to brain bionic eye
The Monash Vision Group (MVG)—a collaboration between Monash University, miniFAB, Grey Innovation and Alfred Health—has taken a different direction, developing a direct-to-brain, or ‘cortical’ bionic eye. Known as the Gennaris bionic vision system, this technology will completely bypass the optic nerve (effectively, it’s a bionic eye system that does not use the eye at all). This makes it suitable for people who have optic nerve damage (a result of glaucoma, diabetes, eye trauma etc). The developers are confident up to 85 per cent of people who are clinically blind could benefit.
Like the retinal-based bionic eye, the technology consists of both internal and external components. Specialised glasses containing a digital camera and movement sensors will capture images, while a small digital processor and wireless transmitter situated on the glasses’ rim will transfer the image the patient is ‘seeing’ to an implant which has been inserted at the back of the brain (directly on the surface of the visual cortex). The implant stimulates the visual cortex via an array of micro-sized electrodes, which create a visual pattern from combinations of up to 473 spots of light (known as phosphenes). Over time, the brain will learn to understand and interpret these signals as ‘sight’.
The Monash Vision Group is planning for first patient implantations to begin by the end of 2016.
In all cases, users of this technology must learn to understand and interpret these light flashes and visual patterns—a process which can take some time.
What can people with a bionic eye actually see?
It’s easy to imagine people with a bionic eye suddenly having sight better than Superman, or experiencing some sort of ‘Terminator’ vision. But the reality, while exciting if you’ve spent years in the dark, is far less dramatic.
When a patient receives a bionic eye implant (either retinal or cortical), they will not suddenly experience the same level of vision as a person with two healthy eyes. Initially, their vision will still be very basic. They may be able to distinguish between darkness and light, or see flickering light and movement in a pixelated form, akin to black and white low-resolution images. Most likely, the ‘image’ they will see will consist of a series of dozens to hundreds of dots of light, configured in a way that will help them navigate the world around them. This is due to the current limitations of the technology, coupled with the patient needing to ‘retrain their brain’ to understand and interpret the visual input it is ‘seeing’.
What the patient will be seeing is known as phosphene GLOSSARY phospheneperception of a luminous image produced by stimulation other than light, such as pressure applied to the eyeball or electrical stimulation. vision. A phosphene is a perceived ring or spot of light in the visual field. It can be produced via pressure on the eyeball, direct stimulation of the visual system (such as via electrodes) or even by a blow to the head, i.e. ‘seeing stars’. This is essentially the experience of seeing light without light actually entering the eye. As the technology improves and further electrodes are added to each implant, more phosphenes will be generated. This will result in patients being able to distinguish even greater levels of detail.
We think it’s going to be initially perceived as dots of various sizes in the distance…The dots could then be programmed, not just to see images, but also to help: Lighting up the clearest pathways out of a room, recognising household objects, or even turning a room full of blurry colleagues dotted at a conference table into a cast of distinct computer-programmed emojis, so the patient can sort out who’s who. It’s just the beginning, really, of a deeper understanding of learning how to work with human perception.Electrical engineer Arthur Lowery, Monash University
One of the challenges for researchers is that the retina is a complex structure of the eye. It contains a large variety of cells, all responsible for distinct visual information, and all of which respond differently to visual input.
When the retina is electrically stimulated via the bionic implant, it excites all of the cells at the same time. This is different to how these cells react in a healthy eye that is receiving ‘real’ visual input via light. The result of over-stimulation means that patients may see blurred outlines, fuzzy contours, indistinct shapes or they may ‘lose’ visual input if an object is moving too fast. Researchers are continuing to work out how to advance implants to produce improved, more natural vision.
Conclusion
The successful development of a bionic eye has the potential to change lives in a very real, very hands-on way. Restoring even basic sight to those with impaired vision may allow them to become more mobile and independent, and return to them some of the quality of life they lost when their vision disappeared. After years of darkness, imagine being able to once again read or to see your loved ones’ smiles. As the technology improves, this may all be possible. However, as with most scientific breakthroughs, it requires a lot of time and a lot of money to bring it to fruition. The bionic ear is now a reality that has helped many thousands of people; let’s hope the bionic eye is not too far behind.