A cometary encounter: Rosetta, Philae and 67P/C-G
Expert reviewers
Essentials
- The European Space Agency launched the Rosetta probe in 2004 to travel to the comet 67P/Churyumov-Gerasimenko.
- Rosetta went into orbit around the comet in September 2014, and sent the Philae lander to the surface of the comet on 12 November 2014.
- Philae’s landing equipment didn’t work according to plan, and the lander bounced a few times before finally coming to rest on the comet’s surface.
- Philae was able to send back data about the composition of the dust on the comet’s surface (similar to dust found on other comets, but with a few extras not seen before), and about the interior make-up of the comet (a lot of empty space!) before it ran out of battery life and powered down.
- Philae woke up from hibernation as the comet got closer to the sun, but only sent limited messages and has been quiet since July 2015.
- Rosetta detected molecules of oxygen in the tail of 67P/Churyumov-Gerasimenko.
- Rosetta analysed water from the comet and found it to have a different deuterium/hydrogen ratio to water on Earth, meaning the water has a different origin.
Floating through space, in a highly elliptical orbit around the sun, is comet 67P/Churyumov-Gerasimenko. The comet is the 67th periodic (67P) comet found, and was first observed by two Soviet astronomers in 1969. Klim Ivanovych Churyumov spotted the comet in a photograph developed by Svetlana Ivanova Gerasimenko (hence the name Churyumov-Gerasimenko).
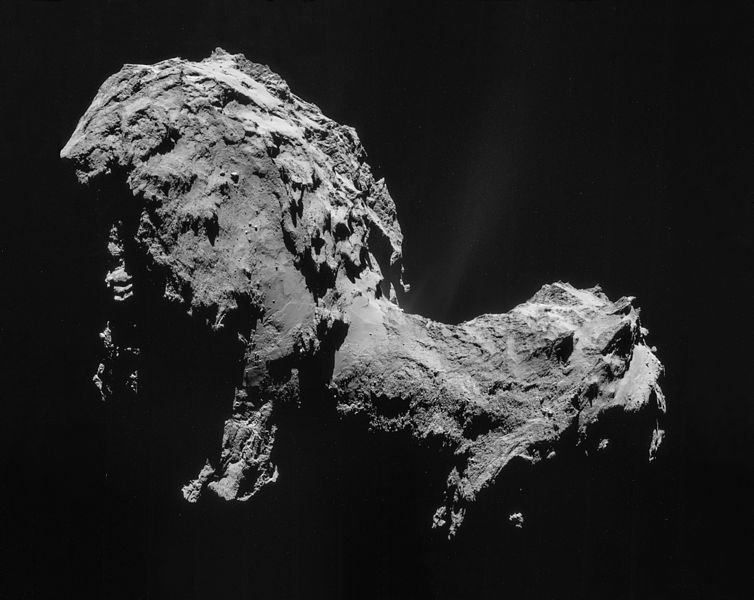
67P is thought to have originated from the Kuiper Belt. This is a collection of dusty ice lumps in space beyond Neptune, and also happens to be Pluto’s home. The Kuiper Belt is around 30–50 astronomical units (one astronomical unit is the distance between Earth and the sun, around 150 million kilometres) away from Earth and it’s thought that all the ‘short period’ comets come from this region. A short period comet has an orbital period of less than 200 years, that is, it takes less than 200 years to complete one orbit around the sun.
Long-period comets, which have orbital periods longer than 200 years, are thought to come from a different location, known as the Oort Cloud. The Oort Cloud is much further away, around 5,000–100,000 astronomical units, right out on the edge of our solar system. The cloud is thought to consist of a trillion (1012) comets. That’s a lot of comets.
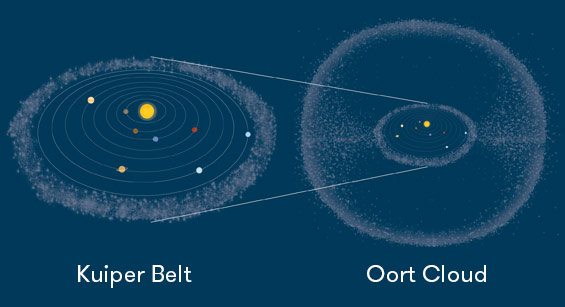
Occasionally, bodies from the Kuiper Belt are pushed out as a result of collisions, or some sort of gravitational disturbance. As they travel into the orbit of Jupiter, the planet’s gravitational influence changes their path. Sometimes they are shunted closer to the sun, and other times they dive into Jupiter itself. Looking at how 67P’s orbit has changed over time, scientists have figured out that the comet’s perihelion (the point in its orbit at which it’s closest to the sun) has been pushed incrementally closer to the sun—before 1840, its perihelion was around 4 astronomical units, but an encounter with Jupiter brought its perihelion to 3 astronomical units. Subsequent encounters brought the comet’s perihelion to 1.29 astronomical units.
The comet has been described as ‘duck-shaped’ with two lobes connected by a narrow neck. Scientists have just figured out that this distinctive shape is the result of a low-speed collision between two separate comets. The smaller lobe is around 2.5 kilometres long and 2 kilometres wide, and the bigger lobe is around 4.1 kilometres by 3.2 kilometres. The comet has a mass of 1013 kilograms. It takes around 6.5 years to orbit the sun.
67P is just one of more than 5,000 comets that have been observed over time. Ancient Chinese Shang oracle bones document sightings of comets, and the Bayeaux Tapestry illustrates the sighting of Halley’s Comet in 1066. Throughout history, their appearance has been thought to be a harbinger of bad luck, or death.
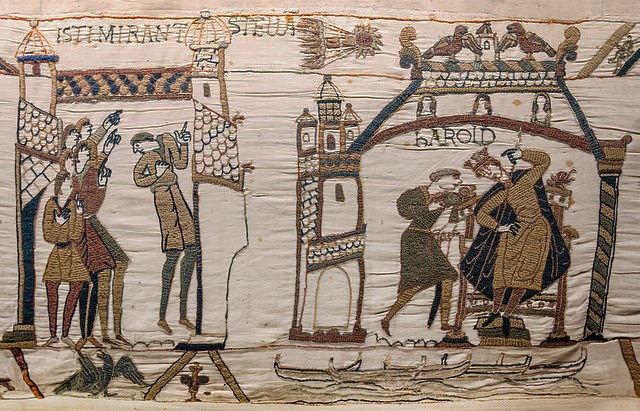
Comets, like asteroids, are thought to be leftovers from the early days of the formation of our solar system. They both orbit the sun, and can have unusual orbits. Being closer to the sun, asteroids’ orbits tend to be smaller and more circular than the often very elliptical orbits of Kuiper Belt comets. Long-period comets from the Oort Cloud are thought to have more circular orbits.
Asteroids are generally made of solid metal and rock. Comets were originally described as ‘dirty snowballs’, comprised mainly of ice mixed with a bit of dust, but a flyby of Halley’s comet by the Giotto spacecraft in 1986 showed the comet was comprised of more dirt than snow. They have icy interiors, with ‘fluffy’ dust layers on their surface.
As comets pass closer to the sun within their obit, some of their ice sublimates—it evaporates directly from ice to vapour, without bothering to become liquid water on the way. The vapour forms a cloud around the comet, called a coma, and it gives the comet a fuzzy appearance. These particles in the coma can interact with cosmic rays from the sun (the solar wind). This excites the particles, which are then converted to ions that trail along behind the comet, in the opposite direction to the sun. These ions form the comet ion tail. Comets often have another tail as well, a long curved tail of dust, made from melted material.
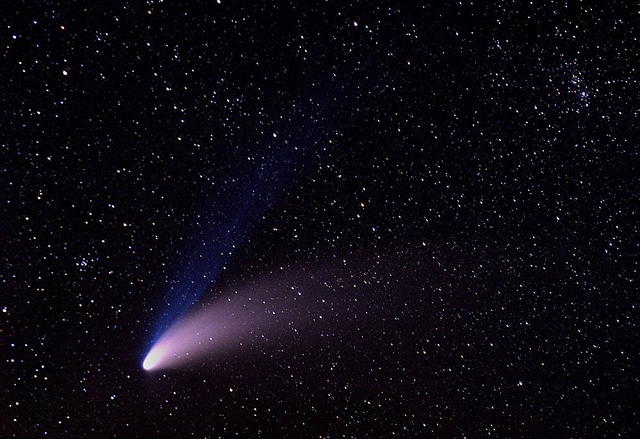
Investigating comets
It’s thought that comets are made from remnants of material that formed very early in the history of our solar system—bits of stuff left over from the formation of the planets. Because they contain a large amount of volatile materials, it’s thought they formed out on the edges of the solar system, away from the sun. Understanding what they’re made of and how exactly they may have formed can offer huge insights into the formation of Earth and the other planets in our solar system.
The Stardust probe was launched in 1999, and in 2004 collected some dust from the coma of Comet Wild-2, using a specially engineered, highly porous, silicon-based sponge-like substance called aerogel. Stardust returned the dust to Earth for analysis in 2006. The material was found to have formed at extremely high temperatures, showing that although comets are primarily icy bodies originating at the cold edges of the solar system, they also contained high temperature material that must have somehow been transported from the inner regions of the solar system to the outer reaches.
In 2005, scientists crashed the Deep Impact probe into comet Tempel 1. Deep Impact blasted a crater in the comet to investigate its insides, creating a huge dust cloud that confirmed it is dirt and dust, rather than ice, that dominates comets’ make-up, as already found by the Giotto mission.
Rosetta and Philae
Nearly 10 years on from Deep Impact and Stardust, the European Space Agency’s (ESA) Rosetta mission has gone one (pretty big step) further. The Rosetta probe was launched on 2 March 2004, and after travelling through space for 10 years, arrived at Churyumov-Gerasimenko on 10 September 2014. Its mission was not to just fly by it, and take some happy snaps and collect some dust as a souvenir, but to actually orbit around the comet, collect tonnes of data (as well as dust!) and to launch the Philae lander down onto the comet’s surface.
Rosetta was named for the Rosetta Stone, a famous slab of volcanic basaltic rock covered in carvings that enabled researchers to decipher ancient Egyptian hieroglyphics, shedding a wealth of new light on this ancient civilisation. The Rosetta mission aims to shed light on Churyumov-Gerasimenko, and comets in general. In doing so, it will help to answer questions about some of the oldest building blocks of our solar system. Philae is named after the island in the Egyptian Nile, where an obelisk was found that contained the final clue necessary to properly understand the heiroglyphics on the Rosetta Stone.
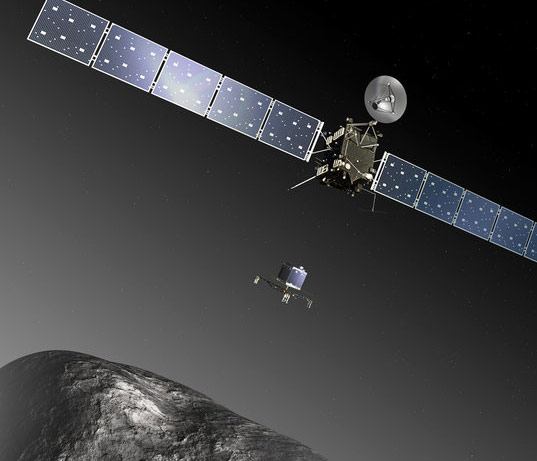
Landing on a comet was a feat of engineering and technological complexity—imagine shooting something around 1 metre across and 80 centimetres high from a spacecraft, to land 22.5 kilometres away on an irregularly-shaped chunk of dirt and ice that’s only around 4.1 kilometres at its widest, with all this taking place 515 million kilometres away from Earth. Advance planning was also a bit tricky as not much was known about 67P’s shape or gravity until Rosetta had got to the comet and conducted some surveys. It was known that what gravity 67P has is very weak, so when the lander touches down, it won’t just stay put by itself. It needs harpoons, retrorockets and ice screws to grab on to the comet’s surface to hold it in place.
12 November, 2014 was the big day. After the Rosetta spacecraft had been in orbit for nearly two months, getting itself into the optimal position, it sent Philae off on its adventure. It took around seven hours for Philae to complete its descent to the comet, and then, travelling at the speed of walking pace (around 1 metre/second), it landed on the comet at the designated landing place, Agilkia … and bounced back off.
A problem with some of the landing apparatus meant that the system set up to hold the lander in place didn’t work, and the lander floated back off the surface, travelling around 1 kilometre from the surface at a speed of around 18 centimetres/second. Churyumov-Gerasimenko’s escape velocity (the speed required to break free of the comet’s gravity and travel off into space) is around 44 centimetres/second and Philae drifted for an hour and 50 minutes before bumping back against the comet’s surface. It drifted again for another seven minutes before finally landing at its ultimate landing place, a dark shadowy spot now known as Abydos, a short distance away from the intended landing place.
Having come to rest in a shady location, where its solar panels wouldn’t be able to pick up much sunlight, Philae had just three days of battery life to do some science before having to go into hibernation. That hibernation lasted around seven months. As 67P got closer to the sun, sunlight was able to recharge Philae’s systems, and Philae woke up again on 13 June. It communicated with Rosetta six times over the next 10 days, sending mainly ‘housekeeping’ data. Another transmission was received on 9 July, but since then Philae has been quiet.
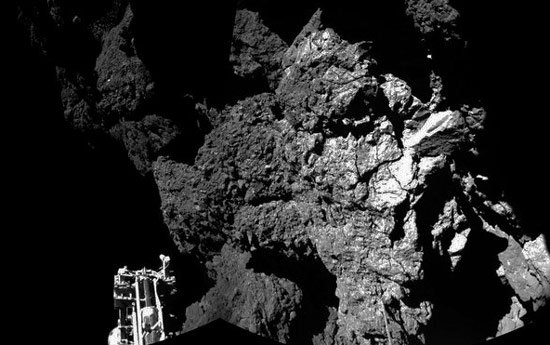
What did Philae find?
Philae is equipped with a range of sensors designed to study various physical and chemical characteristics of 67P.
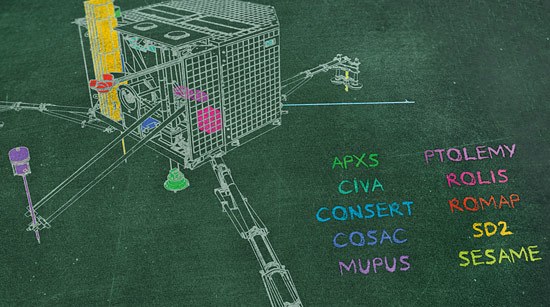
Although there were only three days between Philae’s landing and going to sleep, those three days turned out to be pretty fruitful.
Magnetic field
The extra measurements that Philae was able to make from different locations both on and just above the comet was a silver lining (at least for those studying the comet’s magnetic properties) on the cloud of the unexpectedly bouncy landing.
One of the key findings of the Rosetta and Philae missions has been that 67P does not have a magnetic field. This provides a crucial clue in trying to figure out exactly how comets and other planetary bodies originally formed, showing that magnetism may not have played a role in the formation of small solar system bodies.
More than just dust
Pictures from the CIVA camera show that 67P is home to larger particles than expected—there’s not just dust on the comet’s surface, it’s strewn with pebbles and rocks up to 5 metres across. MUPUS (Multi-Purpose Sensors for Surface and Subsurface Science) information regarding the physical properties of the comet’s surface showed that the surface of the comet is a hard—much harder than expected—dust-rich sintered icy layer.
And the dust contains ...
Although the experiment designed to drill some sample material from 67P’s surface, to be analysed by Philae’s mass spectrometer, didn’t work, scientists were happy to find that some dust that was kicked up when Philae bounced off the surface fell into the sample catcher. Accidental dust is better than nothing!
The material contained organic compounds like those found in the comas of other comets, along with four other types of compounds never before found on comets. Although Rosetta has detected sulphur-rich material just above 67P’s surface, none was found in the dust that was analysed by Philae’s detectors.
Empty space
Analysis of radio waves through the body of the comet conducted when Philae and Rosetta were on opposite sides of Churyumov-Gerasimenko found that its dirt and ice is rather loosely packed, meaning that much of the comet’s interior—77 to 85 per cent—is empty pore space (small areas of space between the particles). There also wasn’t much variation in the insides of the comet, which is surprising, considering the differences that Rosetta has seen in the gases coming out of the comet when its ice sublimates into gas. Unfortunately, there was only time to investigate one end of the comet—so it’s not certain if the other bulgy bit is the same.
What Rosetta did next
Along with receiving communications from Philae, Rosetta also had its own jobs to perform while still orbiting 67P. After the last communication from Philae on 9 July 2015, Rosetta had to shift into an orbit a bit further away from the comet to stay safely away from the increasingly large amounts of dust being spewed forth from 67P as it got closer to the sun. The dust can interfere with Rosetta’s navigation system, which relies on being able to accurately track the surrounding stars to pinpoint its own location. Rosetta moved to an orbit enabling it to explore the southern side of 67P, which meant that it was further away from Philae, sitting on the northern side.
But even before Rosetta deposited Philae onto the surface of the comet, it was making huge discoveries. A question that continues to perplex scientists is where the water and carbon-based molecules essential for life to evolve on Earth first came from. Comets, with their icy composition, were thought to perhaps be responsible for the first, crucial delivery of water to our planet.
Studies of Oort Cloud comets found that water in these bodies has a very different deuterium/hydrogen ratio (D/H; deuterium is a type of ‘heavy’ hydrogen—common hydrogen has one electron and one proton, but deuterium has one electron, one proton and also a neutron in its nucleus, making it slightly heavier) to the water we have on Earth. The comets’ water was ‘heavier’ (that is, had much more deuterium than Earth’s), indicating it has a different origin.
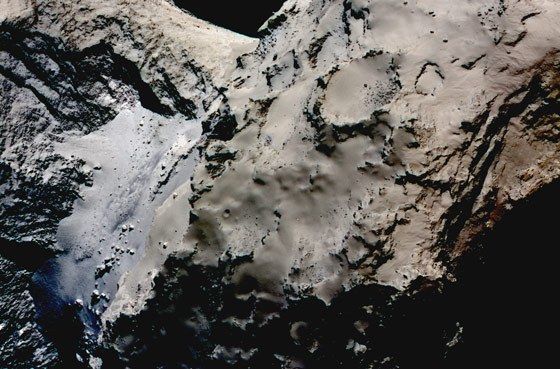
But then, water from the Hartley 2 comet was found to have an identical D/H to the water in our oceans, so scientists thought it was perhaps just the Oort Cloud comets that were out of play, and that maybe the closer comets, like those of the Kuiper Belt, could still be possible water-deliverers.
Rosetta has analysed the water on 67P and found that its D/H is markedly different to Earth’s water—it has even more deuterium than the Oort Cloud comets, once again ruling out comets as the source of Earth’s water.
Another major finding from Rosetta has been the discovery of molecular oxygen—O2, oxygen on its own, rather than in a combination with other elements such as carbon (in carbon monoxide, CO, or carbon dioxide, CO2) or hydrogen (in water, H2O) in the coma of 67P. Both the presence of O2 and the amount of if that’s been found is pretty amazing—oxygen tends to buddy up with other elements pretty quickly.
Scientists studying the discovery believe that the oxygen is still around from way back when the comet formed billions of years ago, trapped in small grains of ice and rock within the comet. But this doesn’t fit with the best current models of how the solar system formed, because according to these, the oxygen wouldn’t have survived—it would have combined with hydrogen. So it’s an intriguing find!
In 2016, after more than 12 years in space, it was time for Rosetta to call it a day. Not only was the spacecraft and its payload getting pretty old but, with the comet moving further away from the sun, the solar power that kept Rosetta’s solar energy systems running properly would soon no longer be sufficient. In late September, commands were sent to the probe to ensure that Rosetta's transmitter and instruments would be turned off. At the end of that month, Rosetta’s mission finally came to an end as it made a slow, controlled landing on 67P—but not before snapping a final picture of the comet, just 20 metres from its surface.