More food, cleaner food—gene technology and plants
Expert reviewers
Essentials
- The physical properties of an organism are determined by its genes
- Gene technology involves manipulating an organism’s genes to enhance or remove particular qualities/characteristics
- Gene technology has the potential to improve crop yields and nutritional value
- There are some potential risks involved with genetically modified organisms, but with the correct oversight, these can be managed
An Austrian monk named Mendel was the first to understand the basic principles by which genes are transmitted from parents to offspring. He worked this out in the 1860s by studying garden peas and the patterns of how certain characteristics (like seed and flower colour) were passed on through the generations of pea plants. Many scientists in the early 1900s further advanced our knowledge of gene transmission. In the following decades, scientists discovered the molecular nature of genes and their products. This led to a revolution in biology which is still gaining momentum.
Producing better crops
Using selective breeding techniques, crop plants and livestock were bred for desired qualities long before people knew anything about the science of genetics. Early plant and animal breeders selected good varieties and strains and bred strong individuals to produce strong offspring without really understanding the underlying genetics. However, now that we do have that understanding, traditional breeding methods have been refined and accelerated.
New varieties of plants are always needed. For example, diseases are continually arising in new forms which can attack previously resistant crops. Plant breeders need to be one step ahead of the pathogens and prepare new resistant varieties for release.
Gene technology provides a new tool
Traditional methods of selective breeding have been provided with several new tools including gene technology. We now have the potential to manipulate the actions of a gene or to take a gene from one organism and move it into another.
Gene technology not only gives us the potential to select the exact characteristics we want in an organism, but it also enables us to cross species barriers. For example, we can take an insecticide-producing gene from a bacterium and insert it into a plant. This plant will then produce that insecticide itself, making the plant resistant to some pest insects. This new-found ability to cross species barriers is what makes gene technology such a powerful tool.
Uses of gene technology
Producing enough food for the world's population without using up all the available land is an enormous challenge. One solution is to develop crops that yield more with fewer inputs; that are more resistant to diseases; that spoil less during storage and transport; that contain more useful nutrients; and that can grow in agricultural land that has been degraded. Gene technology is one of the tools that gives us the potential to do this.
Some Australian examples of gene technology
Insect-resistant cotton
In Australia, caterpillars of Helicoverpa armigera can strip cotton plants of their leaves and nibble their flower buds. The bud develops into the cotton fruit containing the seeds on which the cotton fibres grow, so loss of buds means less cotton is produced. The usual way to control this pest was to spray the plants with chemical pesticides, but now over 90 per cent of Australia’s cotton crop is genetically modified to resist this insect pest. The genetically modified cotton plant produces a protein that, while harmless to other insects, is poisonous to the caterpillar, and so prevents any serious damage to the developing flower buds. The information for making the protein comes from an additional synthetic gene, derived from a bacterium that has been added to the plant. GM cotton plants require far less insecticide than standard varieties of cotton. Pesticide use has been reduced by up to 80 per cent where the GM cotton is grown.
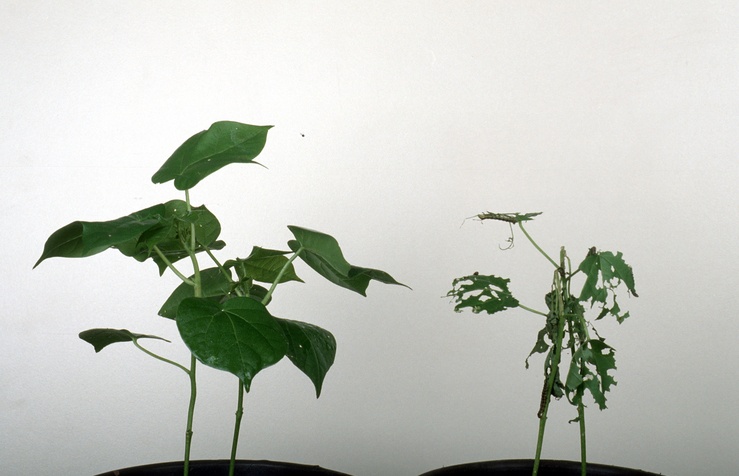
Podborer-resistant cowpea for Africa
Cowpea (Vigna unguiculata Walp.) is a drought-tolerant, heat-adapted annual herbaceous legume. It can be cultivated in areas with very low rainfall. Cowpea thrives in various regions in Africa that have poor soils and erratic rainfall. It is a subsistence crop for tens of millions of rural people, providing essential nutrition as well as income derived by selling the crop in local markets. Most of the world’s cowpea is produced and consumed in Africa.
Cowpea yield can be as high as 1500–2000 kg/hectare, but farmers typically only manage to achieve yields of around 150–400 kg/hectare. The main reason behind these low yields are the problems caused by insects that attack the cowpea crops. Yields can increase by 20 times when insecticides are used, but insecticides are not a good option for this region, as they are expensive, often of low quality, not approved for use on cowpea, and farmers often lack the knowledge and equipment to use them safely.
Australian researchers at CSIRO in collaboration with their colleagues in Nigeria, Burkina Faso and Ghana, with the help of the African Agricultural Technology Foundation (AATF) have produced cowpeas containing the Bt gene, giving them their own built-in resistance to one of the major cowpea pests, the podborer. At this stage the African cowpea breeders are producing farmer-preferred varieties adapted to local conditions and are projected to be released by 2017.
DHA canola
Researchers at the CSIRO Food and Nutrition Flagship have developed canola plants that produce DHA (docosahexaenoic acid), a healthy omega-3 oil vital for human health. DHA is normally only available from fish sources which are declining worldwide. The breakthrough is an important first step towards improving human nutrition, reducing pressure on declining fish stocks and providing Australian grain growers with new high-value crops.
Plants to produce industrial compounds
With the support of the Grains Research and Development Corporation, CSIRO scientists are exploring the potential of plants to make compounds for a variety of industrial uses. More plastics, paints and even nylons could be made from chemicals produced in plants, an environmentally friendly replacement for non-renewable and increasingly costly petrochemicals currently used for the job.
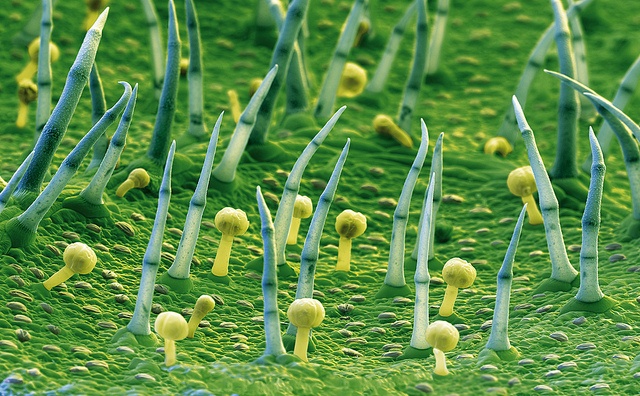
Golden Bananas for Africa
The Highland or East African cooking banana, which is chopped and steamed, is a staple food of many East African nations. However, it has low levels of micronutrients, particularly pro-vitamin A and iron. Vitamin A deficiency is responsible for the deaths of 650,000–700,000 children world each year and it leaves at least another 300,000 blind. Vitamin A deficiency also leads to an impaired immune system and can have an impact on brain development.
In a significant biofortification project similar to Golden Rice, scientists at the Queensland University of Technolgoy Centre for Tropical Crops and Biocommodities, backed by the Bill and Melinda Gates Foundation, are working to fortify bananas with pro-vitamin A. QUT scientists have introduced genes to increase the level of pro-vitamin A in the bananas, which will significantly improve the health of the people who rely on the banana as one of their staple foods.
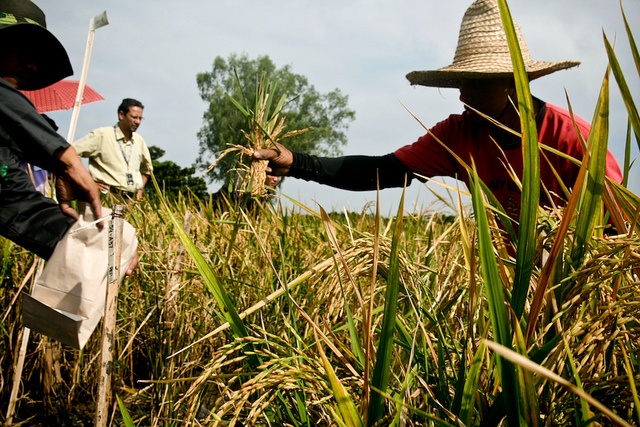
Workers and scientists collaborate during a confined field trial of Golden Rice, which is genetically modified to include vitamin A. Image credit: International Rice Research Institute on Flickr.
Potential benefits; potential risks
Gene technology promises increased yields and reduced dependence on pesticides. However, as well as potential benefits, there are also potential risks.
Pesticide resistance
It is now possible to genetically engineer plants that produce their own pesticide. This exposes pests to the pesticide every day rather than as burst of pesticide application by the grower. If the pesticide-producing capability is introduced into a number of different plant species, it could accelerate the development of pesticide resistance among pests. (To reduce this concern the US Environmental Protection Agency has restricted the sales of pesticide-producing corn to states that do not grow pesticide-producing cotton.)
Increased use of herbicides
When farmers spray a herbicide to remove weeds growing among crops, the sprayed chemical often damages the crop plants. If the crop is engineered to be resistant to the chemical, the weeds will be killed but the crop plants will remain undamaged.
At first glance, this seems like a good thing. But it is likely to lead to greater use of the particular herbicide, which could have one or more negative effects:
- the crop could contain greater herbicide residues
- the increased spraying will contaminate the rest of the environment
- the repeated spraying of one herbicide is likely to result in herbicide resistant weeds
Of course, not all herbicides are dangerous, but it is safer to use them at their correct dose and to rotate between different types rather than encourage the repeated use of any one type.
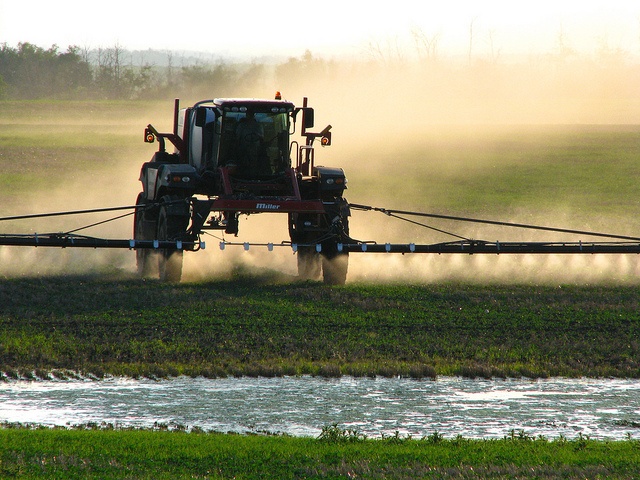
Herbicide-resistant weeds
Genetic engineers are producing crops that are herbicide-resistant and pesticide-resistant. If the genes for these characteristics were to end up in a weed species, the weed would thrive and be difficult to control. (Field trials in Denmark of a genetically engineered, herbicide-resistant canola showed that the gene for herbicide resistance had jumped into a closely related plant.)
To label or not to label
One of the main points of controversy surrounding the release of genetically modified organisms is the question of labelling food products. Supporters of labelling point to potential problems for people with food intolerances. An investigation carried out in the mid-1990s found that seven out of nine people allergic to brazil nuts were also allergic to soya beans that had been genetically modified to contain a protein usually found in the nuts. These people showed no reaction to unmodified soya beans, so the protein taken from the brazil nuts must have been responsible for their allergic reaction. Because serious food reactions can kill, people need to know when genetically modified products might cause allergic reactions.
Supporters of labelling also point to the principle of the consumer's right to know what is in their food. Opponents point out that we don't know exactly what is in our food at the moment anyway. Most plants contain natural toxins to protect them against insect attack, and at high concentrations these toxins are certainly not good for us. However, we are generally happy to eat a parsnip, even though they contain potentially carcinogenic (cancer-causing) chemicals, safe in the knowledge that the concentration of the naturally occurring carcinogenic chemical is low enough that it won’t harm us.
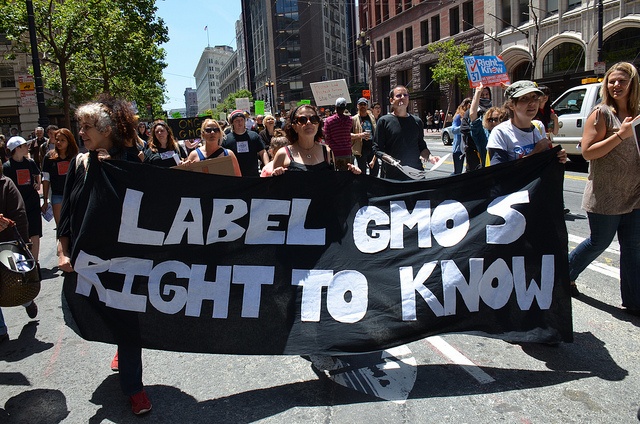
Concerns about marker genes
Other worries stem from the use of marker genes. These are genes that are inserted into the genetically modified organism along with the desired gene. The presence of marker genes, which are easy to spot, allows researchers to recognise organisms that contain the desired gene. The problem arises with those marker genes that also give antibiotic resistance to the organism.
Some people believe it is risky to allow genetically modified plants with marker genes for antibiotic resistance into the environment. It was feared that the gene for resistance to kanamycin (a common antibiotic drug) in plants could spread to bacteria that inhabit the human gut. In turn, these could pass the gene on to more dangerous bacteria. Or that the marker gene could move from the plant into soil bacteria and then into disease-causing bacteria. Extensive research showed that there was a negligible risk of this occurring and the European Food Safety Authority (EFSA) concluded that there was no scientific evidence to preclude use of such a marker gene.
The role of big business
It is expensive to develop the potential that gene technology offers and it requires a long-term financial commitment to research. While large and well-funded corporations are able to provide this amount of money, there is some concern that the results of this research will not be readily accessible to small companies, government-funded organisations or countries with emerging economies. Some people feel that it is not wise to place the responsibility for the world's food supply into the hands of a few large companies. However, opposition to the use of gene technology by multi-national corporations, has inadvertently made it near impossible for the public sector to afford the deregulation costs of its discoveries.
Tampering with nature
Critics of gene technology suspect that we still know too little about the systems that we are tampering with. Could an inserted gene have effects that we are unaware of? Could it upset the balance of existing genes, causing the plant to produce greater quantities of natural toxins, or to change its nutritional content?
Most researchers argue that there is no evidence of such unexpected changes. They point out that gene technology is much less likely to have unwanted effects on a plant than traditional selective breeding methods. These traditional methods, which have been carried out for thousands of years, involve the movement of thousands of genes from one organism to another. Modern gene technology, on the other hand, moves only a few targeted genes.
Government safeguards
So, as with all new technologies, we must ensure that we proceed carefully. Before genetically modified organisms are released into the environment, there is usually a prolonged period in which they are kept within a contained laboratory. Initial testing in a more open environment is then closely monitored and restricted. In Australia, both lab-based testing and field trials are overseen by the Office of the Gene Technology Regulator (OGTR). The OGTR provides administrative support to the Gene Technology Regulator, who is responsible for enforcing the Gene Technology Act 2000, ‘a national scheme for the regulation of genetically modified organisms in Australia, in order to protect the health and safety of Australians and the Australian environment by identifying risks posed by or as a result of gene technology, and to manage those risks by regulating certain dealings with genetically modified organisms’ (OGTR website).
‘Dealings’ include research, production, manufacture, import, storage, transport and disposal of genetically modified organisms (GMOs). To release a GMO into the environment, the Regulator prepares a risk assessment and risk management plan by consulting scientific experts, stakeholders and the public. The Regulator then decides whether or not to issue a licence to allow the release of a GMO.
Another regulatory body is Food Standards Australia and New Zealand (FSANZ), which oversees genetically modified organisms that end up as human food They develop food standards for composition, labelling and contaminants that apply to all foods produced or imported for sale in Australia and New Zealand.
The Australian Pesticides and Veterinary Medicines Authority (APVMA), which regulates agricultural and veterinary chemicals, including herbicides and pesticides. It is responsible for genetically modified pesticide-resistant plants.