Zap! How to make a REALLY intense laser beam
Lasers. We use them for communications, surgery, law enforcement, entertainment, printing, engraving, measuring, researching … the list of applications is seemingly endless.
They’ve been on the scene since the early 1960s when researchers first started theorising and experimenting with compressed, controlled beams of light energy. During the first few years since their invention, development of more powerful pulsed lasers continued rapidly.
But then researchers hit a wall. The powerful lasers they were trying to build had a nasty habit of damaging the machinery being used to generate them. Progress stalled for over a decade. Then, in the mid-1980’s, Donna Strickland and Gérard Mourou came up with a new way to make lasers that changed everything … and in 2018, their work was recognised with a Nobel Prize for Physics (alongside Arthur Ashkin’s development of ‘optical tweezers’ – more laser physics).
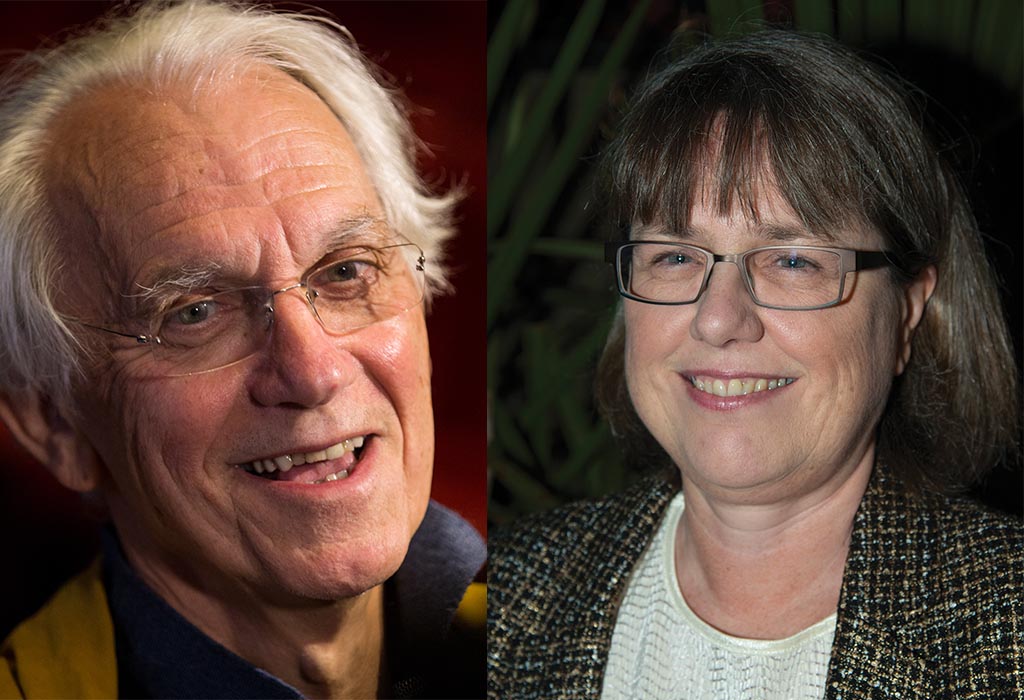
So what makes this invention so important—to the point of being worthy for a Nobel Prize?
Lasers basically work by getting a bunch of atoms all excited. You can ‘excite’ an atom with a flash of light, through causing collisions between atoms, or by supplying electricity.
An ‘excited’ atom has absorbed enough energy to jolt it from its normal resting ‘ground’ state into a higher-energy ‘excited’ state. Excited atoms will naturally want to return to their normal lower-energy ‘ground’ state. In the process, they’ll spontaneously release their extra energy by emitting a particle of light called a photon.
If you send even more energy towards an already-excited atom, it jumps back to a lower-energy state, but releases two identical photons with the same colour, level of energy, and direction of movement. This process (called ‘stimulated emission’) allows you to increase the intensity of light. Bouncing the light between two carefully-aligned mirrors will drive a chain reaction of excited atoms exciting other atoms, triggering the formation of a cascade of identical photons and amplifying the light more and more. By letting some of that light escape on one side, we end up with light that consists of just one colour, travelling in one direction in a narrow, focused beam: a laser.
Laser light is different to the light you get from a regular old lamp or an LED: all the waves of light run parallel to each other, rather than scattering in all directions; there’s only one specific colour and wavelength; and it can be fired as a series of short, intense pulses.
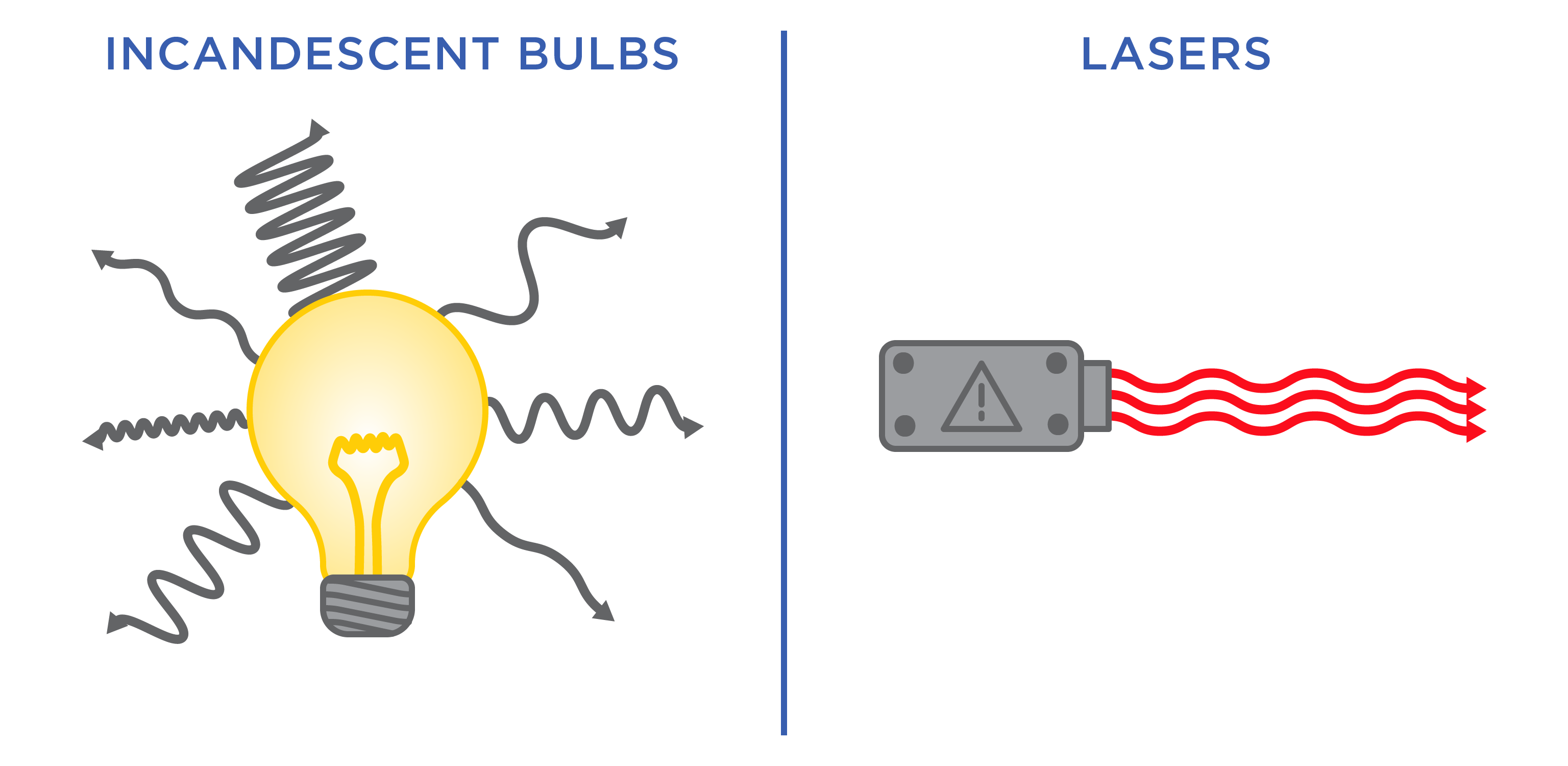
The more energy you pump into the laser to get those atoms all excited, the greater the intensity of the light. It’s a little like turning up the volume dial on a sound system. Turn that volume up too high, though, and you risk causing damage (either to the sound system itself, or to your hearing!).
This is the problem researchers faced before Strickland and Mourou’s discovery: as they increased the amplification of their lasers, they reached a point where the intensity of the short, sharp pulses of light with their bunched-together photons was too much, damaging the amplification equipment itself.
The solution was to modify the length of the pulses of light energy so that the intensity was spread out more evenly as it moved through the amplifier. The technique, called ‘chirped pulse amplification’ (CPA for short), involves three main steps: stretch a short light pulse out in time and space, amplify it, then compress it again.
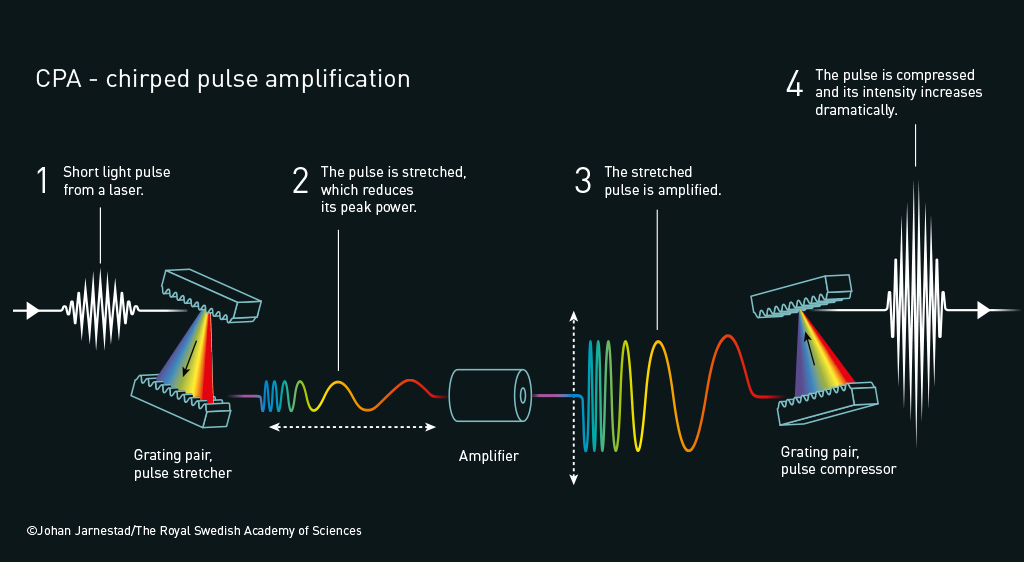
Stretching the pulse in time reduces its intensity. Different components of the pulse reach different parts of the amplifier at slightly different times, spreading out the load and reducing the damage.
As the pulse travels through the amplifier, it’s time to turn up the volume and make the pulse bigger. This produces a pulse that is still stretched out in time but with its power increased significantly.
The final step is to take all of that boosted power and compress it outside the amplifier back into a short, sharp, fast burst of light. All of that amplified energy is packed into a small space and short pulse, upping the intensity yet again. The result is a laser pulse that packs a real punch.
This breakthrough opened up several new areas of research in laser physics and a whole bunch of new practical applications. A very intense, very short laser pulse allows for extremely high precision on very small scales. High-quality devices such as the stents doctors insert into arteries, or some of the components in a smartphone, can only be produced with the ultra-short pulses of laser machining.
Extreme precision on a tiny scale is also a very welcome development in the field of laser eye surgery. Thanks to developments in laser physics that were kick-started by Strickland and Mourou’s work, it is now possible to carefully sculpt and shape the cornea (the transparent layer at the front of the eye) with minimal invasiveness, improving the vision of millions of people around the world every year.
Ultrashort pulses also allow us to study events taking place at the level of individual atoms, and the emerging field of attosecond physics (an attosecond is one billionth of a billionth of a second) takes us even further in being able to observe and control individual electrons around those atoms.
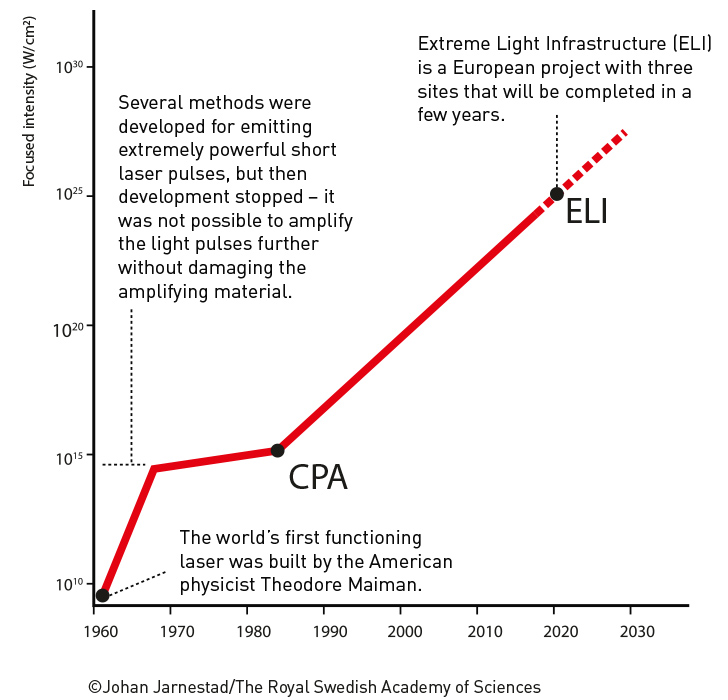
What else will be possible in the future with extremely intense, fast pulses of light? There’s a long list of potential applications, both for research and industry. Gérard Mourou and Donna Strickland’s ‘chirped pulse amplification’ techniques have opened the door to harnessing the power of light to measure and manipulate our world: a development well and truly worthy of such high acclaim.