Kissing the Epstein-Barr virus goodbye?
Expert reviewers
Essentials
- Almost 95 per cent of adults carry the Epstein-Barr Virus (EBV)
- EBV causes glandular fever, also known as ‘the kissing disease’
- Depending on the carrier, responses to EBV can range from no symptoms to extreme illness
- EBV is spread orally, primarily through saliva
- Globally, around 200,000 cancers a year are attributed to EBV
- Vaccine trials are underway, but require further research and testing
Listen to this topic
Kissing can sometimes lead to heartbreak, but did you know it can also be hazardous to your health? Pucker up to someone and you risk infection with the Epstein-Barr virus, which causes glandular fever (also known as infectious mononucleosis, or colloquially as ‘the kissing disease’) as well as a host of other nasty infections.
But we might not have to give up kissing just yet. A vaccine currently under development at an Australian laboratory, the QIMR Berghofer Medical Research Institute, could help limit the dangers posed by this sometimes deadly virus.
What is the Epstein-Barr virus?
The Epstein-Barr virus (EBV) is one of eight viruses in the herpes family. Also known as human herpesvirus 4 (HHV-4), it is one of the most common viruses in humans, with between 90 and 95 per cent of adults infected.
While it is best known as the cause of glandular fever, it is also associated with a wide range of more serious illnesses, including
- Cancers—such as Hodgkin’s lymphoma, Burkitt’s lymphoma, nasopharyngeal carcinoma, some stomach cancers and possibly breast cancer
- Conditions associated with Human Immunodeficiency Virus (HIV)—including hairy leukoplakia and central nervous system lymphomas
- Auto-immune diseases—dermatomyositis, systemic lupus erythematosus, rheumatoid arthritis, and multiple sclerosis
It is estimated that every year, around 200,000 cancers are directly attributable to EBV.
At present there are two known strains of the virus, EBV-1 and EBV-2. EBV-2 is less effective at causing B-cell growth and proliferation than EBV-1. Scientists don’t know why the virus causes a relatively mild disease like glandular fever in some people and malignant tumours in others. Some evidence suggests that genetic factors and reduced immunity GLOSSARY immunityA body's reaction to the introduction of foreign substances, through the production of defensive substances such as antibodies. may play a role.
How it was discovered
In 1961, a surgeon working in Uganda, Denis Burkitt, presented the results of his research to staff at the Middlesex Hospital Medical School in Britain. He reported that the incidence of a certain tumour in African children had a geographic distribution corresponding to rainfall and temperature patterns.
The disease, which affects about 8 in every 100,000 children in parts of Africa and Papua New Guinea, quickly became known as Burkitt’s lymphoma. The influence of climate on its incidence seemed to suggest that some biological factor was involved. Three researchers, M.A. Epstein, Y.M. Barr and B.G. Achong, immediately began looking for possible cancer-causing viruses in samples of the tumour sent from Uganda to Britain.
In 1964, they identified the culprit using an electron microscope: a previously unknown member of the herpes family of viruses. Epstein and Barr were awarded the dubious honour of having the pathogen named after them. It was the first virus to be directly associated with human cancer.
How the virus spreads
EBV is spread mainly via the transfer of saliva between individuals, which is the reason that glandular fever has been dubbed the ‘kissing disease’. It can also be spread by sharing utensils/toothbrushes etc, through blood (transfusions, organ transplants) and rarely via semen from sexual contact. People infected with the Epstein-Barr virus will retain it for life, but it may not make them sick. In fact, the virus infects almost everyone in developing countries and more than 95 per cent of people in developed countries.
Most people are infected with the virus during childhood, probably by their mothers, and are usually not noticeably affected. On the other hand, people infected for the first time during or after adolescence (10‑20 per cent of people living in developed countries) have a 50 per cent chance of contracting glandular fever. They may be ill for several weeks or months before their immune system kicks in to action.
Symptoms of EBV
The symptoms of EBV can include:
- fever/chills
- fatigue
- inflamed throat
- swollen lymph nodes in the neck
- swollen liver or spleen
- rash
- loss of appetite
- minor aches and pains
Often the symptoms of EBV are not distinguishable from other general colds and flu. Those who do experience symptoms generally recover within 2 to 4 weeks, however full recovery can take months. It is important to note however that while your symptoms may have disappeared, the virus has not. Instead it becomes inactive—essentially hiding away inside the body’s B-cells. It is possible for the EBV to ‘reactivate’ later in life, though this is rare.
Diagnosing EBV
It can be difficult for doctors to diagnose EBV because its symptoms so closely resemble a range of other illnesses. However, a blood test can confirm the presence of EBV antibodies in the system, whether someone is susceptible to the virus, or has had a recent or past infection. Find out more about the laboratory testing of EBV.
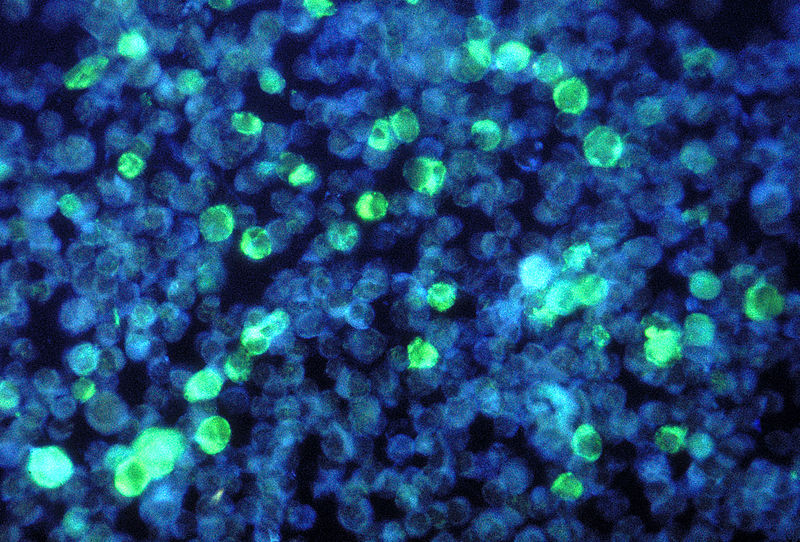
A photomicrograph showing cells containing the Epstein-Barr virus. Image source: CDC/Dr Paul M. Feorino / Wikimedia Commons.
How does the virus work?
EBV produces about 100 different antigens GLOSSARY antigens A toxin or other foreign substance, usually a protein, which induces an immune response in the body, especially the production of antibodies. (large protein molecules) during the active phase of the viral cycle. In contrast, only about 10 antigens are produced during the inactive phase: these include the EBV nuclear antigens (EBVNAs 1–6), and the latent membrane proteins (LMPs 1–3).
EBV primarily infects the oropharynx (the part of the throat at the back of the mouth), specifically the salivary glands, as well as the oral mucosal membrane (the mucous membrane lining the inside of the mouth) and the nasopharyngeal epithelial tissue (above your soft palate). The virus replicates quickly in these areas, enabling it to spread easily through saliva from one person to another.
But EBV doesn’t stop there. After the initial infection it begins to infect white blood cells known as B lymphocytes (B-cells). Infection of B-cells with the virus transforms them into B-cells with unlimited growth potential, causing them to rapidly multiply and increase in number. This proliferation is combated by the immune system; it activates Cytotoxic T lymphocytes (T-cells) and Natural Killer Cells in defence against EBV, which combine with certain antigens produced by the virus and kill cells that harbour it.
Unfortunately, when the virus is associated with Burkitt’s lymphoma and nasopharyngeal carcinoma it appears to produce only one antigen—EBNA1. T-cells are unable to combine with this particular antigen and will not attack the infected cell.
Although our immune system may seem to have beaten EBV, in reality it has been duped. When our immune system begins to attack EBV, the virus admits defeat and retreats into hiding, lurking dormant inside the body’s B cells. In this way it continues to live ‘under the radar’. It is no longer under threat from the immune system, but is able to keep spreading from person to person without our knowledge that it’s even there. Occasionally if the correct conditions exist in the future (such as reduced immunity through infection), the virus can sometimes reactivate.
In some people, however, the T-cell response is not strong enough, resulting in unrestricted growth of B-cell lymphocytes, which greatly increases the risk of the patient developing a form of cancer.
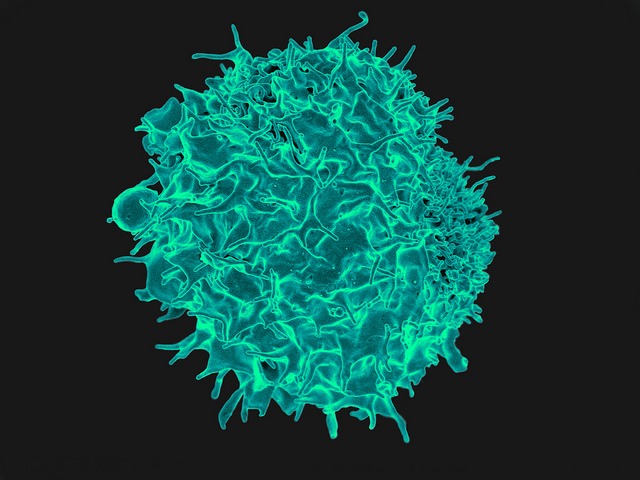
A colourised electron micrograph image of a T-cell, one of the body's defences against EBV. Image source: NIAID / Flickr.
How does EBV induce the growth of cancers?
Since its initial discovery as the first human cancer virus, EBV has continued to be associated with the development of a wide range of tumours. Scientists have been working for years to determine why EBV causes cancer in only a small percentage of the vast number of people who carry the virus, how it develops into different types of cancers, and why it affects different populations and age groups across the world.
A key piece in the puzzle was understanding how our immune systems respond to and fight EBV. In the 1970s doctors discovered that a large number of patients who had received transplants in the decade before had developed cancer—generally skin cancer but also lymphomas too. These became known as post-transplant lymphoma, but doctors could not work out what caused them. Samples of these tumours were tested and found to be positive for EBV.
With the outbreak of the AIDS virus in the 1980s, it was found that some patients of the disease developed certain types of cancer including lymphomas that had close similarities to post-transplant lymphomas. When analysed, almost all the samples tested positive for EBV too.
What was the link between people with HIIV and transplant patients? The answer lay in their immune systems—specifically its weakened state after either transplant or fighting the AIDS virus.
Further understanding of the virus and the way it worked came in 1984 when the complete DNA sequence of EBV was published. The key proteins made by the virus—those which allowed it to push B cells to multiply and become cancerous—were now known to scientists.
Researchers concluded that although there were a combination of variables that caused EBV cancers to develop, there was one consistent factor—a change in the balance between the immune system and the virus. Basically, the ability of the body and its immune system to stay in control of the virus was imperative to stopping cancers forming.
A weakened or poorly functioning immune system (due to illness, disease, drugs or even a genetic variation in genes that reduces the ability of immune cells to spot EBV) result in the normally shy and contained virus kicking up its heels and running rampant. The proteins made by the virus direct the cells to keep dividing and multiplying exponentially—the principal cause of cancer.
The search for a vaccine
As with most viruses, the best chance of defence against EBV is vaccination. Yet a vaccine has been elusive, partly because the virus is so good at hiding.
To prevent Burkitt’s lymphoma or nasopharyngeal carcinoma, a vaccine would need to provide 100 per cent immunity or be capable of establishing a T-cell population that recognises EBNA1. Both tasks are extremely difficult. It may be possible, though, to produce a vaccine against glandular fever and post-transplant lymphoproliferative disease, since both produce antigens that are recognised by T-cells.
Australian scientists have produced such a vaccine. Based at the QIMR Berghofer Medical Research Institute, they have conducted a trial with a prototype vaccine using human volunteers.
One of the first steps in the development of the vaccine was to define the antigens of the virus that are important in the immune control of it. Antigens stimulate the manufacture of T-cells; if they—or their most important parts—could be introduced artificially to an individual, then the immune system might be ‘tricked’ into producing T-cells that would recognise the real antigen should infection occur.
The researchers were able to produce a peptide identical to part of the EBV antigen EBNA3. This peptide formed the basis of the vaccine: by injecting it into patients, researchers hoped to ‘arm’ the body with T-cells in readiness for an invasion of the real disease.
The trial has been completed and scientists are now confident that the vaccine does not have any harmful effects on patients. Recipients didn't develop infectious mononucleosis, but being a small trial the vaccine's effectiveness in preventing disease could not be accurately assessed.
Another vaccine developed in Europe that is based on a surface protein of the virus, gp350, has successfully reduced EBV infections in an 18-month trial. Attempts are also being made to grow and expand T-cells in the laboratory to help cure various forms of EBV-induced cancers. This approach should be particularly useful for treating patients suffering from post-transplant lymphoproliferative disease.
Recently, a group from Germany described an alternative strategy for EBV vaccine. This group produced non-infectious virus-like particles in which several EBV proteins critical for viral pathogenesis GLOSSARY were either functionally inactivated or deleted. Immunisation of animals with these virus-like particles induced anti-EBV neutralising antibody and cellular immune responses. Human trials with this vaccine formulation are expected to start very soon.
Over the last decade, there has been considerable progress on the development of therapeutic vaccines for EBV-associated cancers. These diseases include nasopharyngeal carcinoma, Hodgkin lymphoma, non-Hodgkin lymphoma and T-cell lymphoma, which generally express limited number of EBV proteins.
Two different types of therapeutic vaccines have been tested in patients with nasopharyngeal carcinoma. One of these vaccines is based on a poxvirus vector (modified vaccinia Anakara) which expresses EBV encoded EBNA1 and LMP2 proteins. Nasopharyngeal carcinoma patients in Hong Kong who were immunised with this vaccine showed and a three to fourfold increase in the magnitude of T-cell responses to the proteins. Similar results were also obtained from another follow-up study in the United Kingdom. Another vaccine is based on dendritic GLOSSARY dendritichaving a branched form resembling a tree cells sensitised with EBV peptides or infected with viral vectors encoding EBV proteins. This vaccine induced immune response against the EBV proteins and tumor regression in two of nine patients.
Conclusion
Despite its prevalence in the human population, finding a cure for EBV has not been seen as a high priority. This may be because the majority of people do not suffer serious health effects, while those who do tend to live in developing countries. However, as new cancers are linked with the virus, the need to find a solution will become of more interest to researchers, drug companies and pharmaceuticals. And that would certainly be worth a celebratory kiss.