Stem cells—sorting the hype from the reality
Expert reviewers
Associate Professor Megan Munsie
Head, Education, Ethics, Law & Community Awareness Unit
Stem Cells Australia
Essentials
- Most of the human body’s 37 trillion cells have a specialised function e.g. liver cell, brain cell, heart cell
- Stem cells are ‘immature’ types of cells that have not yet developed their specialisation
- Stem cells can divide to replicate themselves/make more of themselves
- There are two main types: tissue stem cells and pluripotent stem cells
- Stem cells are crucial for healthy development and for maintaining the healthy function of our body’s systems
- Stem cell treatments have the potential to help the body repair and replenish injured or damaged tissue or organs by producing new cells to replace the damaged ones
- Stem cells are a tool for studying disease and development
Our bodies contain around 37 trillion cells, of which there are hundreds of different types—liver cells, skin cells, hair cells, muscle cells, brain cells, to name just a few. These cells are specialised—they have a specific function within the body. A brain cell cannot do the job of a skin cell.
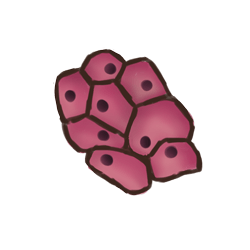
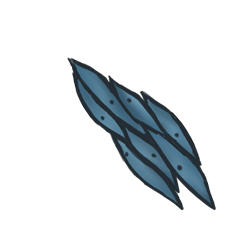
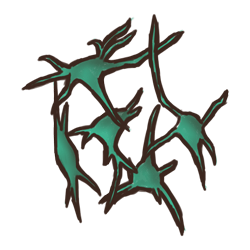
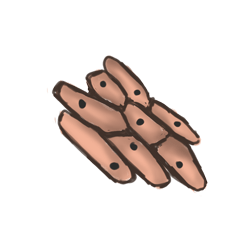
There is another type of cell—stem cells—which are an ‘immature’ type of cell, that have not yet developed a specialised function.
Stem cells
There are two very important things about stem cells:
- they have the ability to divide and replicate themselves, to make more stem cells (self-renewal)
- they can become adult specialised cells through a process called differentiation—they might go on to become a liver cell, a heart cell or a muscle cell
Most of our body’s organs contain stem cells.
Tissue-specific stem cells
Some stem cells have limited options. They can usually only develop, or differentiate, into cells to replenish the organs in which they are found, replacing cells that are lost or die because of injury or disease. They also aid homeostasis GLOSSARY homeostasisThe process of healthy growth and development, and maintenance of healthy functioning of an organism. in healthy tissue. These stem cells are known as tissue-specific, adult, or somatic stem cells.
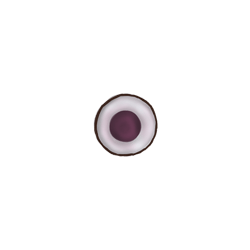
Some organs, like our skin, have highly active stem cells—we’re constantly rubbing away at our skin and shedding skin cells, so it's important to replace the cells we lose. We are also constantly shedding cells from our gut, so we need stem cells there to produce new cells to maintain normal function.
Stem cells are also present in other organs, like our hearts or brains, but their regenerative capacity is more limited. Understanding why the stem cells in these organs do not respond in the same way in response to injury or disease is the focus of current research.
Pluripotent stem cells
For some stem cells, the world is their oyster. Pluripotent stem cells can turn into any one of the many specialised cells—they are not restricted to any one particular type of organ or tissue.
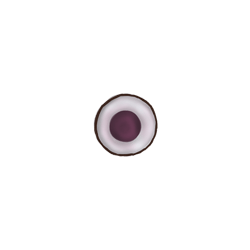
There are two types of pluripotent stem cells:
- embryonic stem cells (ES cells)
- induced pluripotent stem cells (iPS cells)
The capacity to become anything—embryonic stem cells
After an egg is fertilised, it starts to divide to make new cells. As development progresses, the cells initially differentiate into two different types of cells—the inner cell mass and an outer cell layer called the trophectoderm which surrounds the embryo. This stage of development occurs 5–7 days after fertilisation. The trophectoderm cells will contribute to the placenta, and those of the inner cell mass will develop into a foetus if a pregnancy is achieved.
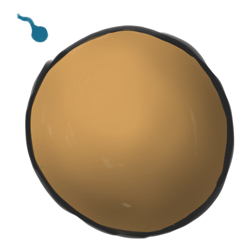
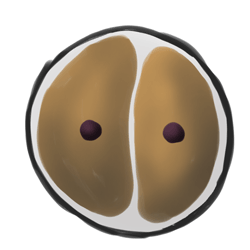
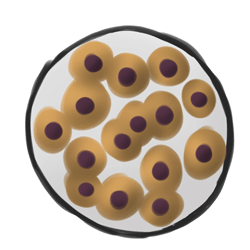
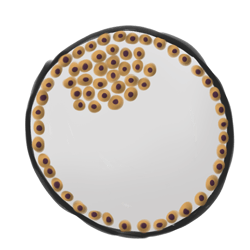
In stem cell research, embryonic stem cells are sourced from the inner cell mass of embryos donated by couples who have undergone infertility treatment. These early stage (pre-implantation) embryos are no longer required by the couple to achieve pregnancy (usually because they have completed their family) and if not donated to research, would most likely have been discarded. In Australia, research using embryonic stem cells, sourced from donations of excess embryos used in IVF procedures, has been legal since 2002. It is strictly regulated and scientists must justify their requirement to use embryonic stem cells to an independent licensing committee.
The art of gentle persuasion—iPS stem cells
Another way to make pluripotent stem cells is by ‘forcing’ or ‘coaxing’ specialised adult cells (like skin cells or blood cells) to revert back to an immature stem cell state. Scientists have found that reintroducing some crucial genes or adding certain proteins to the culture media containing the adult cells can turn back the ‘differentiation clock’, producing pluripotent stem cells capable of self-replication as well as differentiation. These are called induced pluripotent stem cells (iPS cells).
Only discovered in 2006, scientists’ understanding of this process is constantly evolving, and iPS cell technology is still being refined. However, the technique has huge potential to advance stem cell research and scientists around the world are working on developing iPS cells.
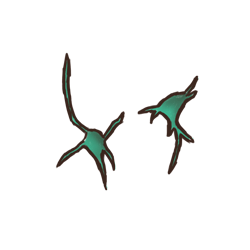
iPS cells have been lauded by some as a solution to the ethical issues surrounding the use of embryonic stem cells. But in reality, it’s not quite that simple. iPS cells come with a range of ethical issues of their own. As they are derived from adult cells, iPS cells will contain all of the donor’s genetic material, making anonymity and privacy a potential problem. Furthermore, it would theoretically be possible to make egg and sperm cells from iPS cells, and then use these to create a new human life. This is pretty firmly in the realm of science fiction at the moment, although researchers have managed to clone mice and pigs from iPS cells. The research community stands united in staunch opposition to developing this sort of technology for human reproduction.
Understanding the process by which an embryonic stem cell or an induced pluripotent stem cell develops into a specialised cell is crucial before scientists can even begin to think about building new organs. Australian researchers led by Martin Pera are looking at how stem cells first start out on the path of differentiation, and also how neural (brain) cells develop. Find out more about the research at Stem Cells Australia.
What we can already do with stem cells
While stem cells certainly offer great hope for future treatments, the current range of proven stem cell treatment in Australia, and indeed, around the world is quite small.
Blood treatments
Blood stem cell (haematopoietic stem cell, HSC) transplants are already routinely used to treat a number of blood and autoimmune diseases, and some cancers, like leukaemia, lymphoma and thalassaemia. The HSCs help the patient’s bone marrow (which makes red blood cells, white blood cells of the immune system and platelets) function properly again. HSCs can be sourced from bone marrow or from umbilical cord blood. In the former, HSC production is stimulated by introducing a growth factor called GCSF into the patient's blood circulation that encourages their growth. This can be time consuming and can have side effects such as bone pain and spleen enlargement for some patients. CSIRO has recently carried out pre-clinical trials on a new method of harvesting stem cells which eliminates the need for the growth factor.
Corneal grafts
Treatments involving stem cells from the cornea have successfully helped people suffering from eye disease or injuries to the surface of the eye. Stem cells from the edge of the cornea, known as the limbus, are taken from the patient and used to grow new corneal tissue in the laboratory. The new tissue is then grafted on to the patient’s eye.
Skin grafts
Using skin stem cells to treat burns has been a common and effective treatment for decades. Using skin stem cells from the patient, new skin is grown in the laboratory and then grafted over the injured area. The process of growing the new skin takes around three weeks, during which the patient may suffer from dehydration or infections. Although the practice of using stem cells to create skin grafts has been around a long time now, it is still being refined. Each new development means limitations can be overcome, and more patients can be successfully treated.
A colony of embryonic stem cells viewed at 10X magnification. Image source: Ryddragyn / Wikimedia Commons.
Treatments in the future
Scientists in the lab are continually redefining the line between scientific reality and science fiction. Almost daily, new results are announced that reinforce the potential of stem cells to revolutionise doctors’ capacity to help patients with serious injuries or diseases.
Several researchers around the world have been able to grow types of tissues dubbed ‘organoids’ from stem cells. These tissues resemble the structure and function of actual human organs. Tiny human intestines have been grown in mice, after researchers from the Cincinnati Children’s Hospital Medical Centre implanted intestinal cells made from iPS cells. A completely functional thymus was grown in a mouse by researchers at the University of Edinburgh, marking the first time a full organ has been grown using stem cells.
Hans Clevers and his collaborators are developing methods to study cancer and other diseases in organoids of gut and intestinal tissues, growing ‘mini-guts’ from stem cells.
A team led by cardiologist James Chong including researchers from the University of Sydney, Sydney’s Westmead Millennium Institute and the University of Washington has managed to regrow damaged heart tissue using human embryonic stem cells. The stem cells were injected into monkeys that had suffered heart attacks, and 40 per cent of the damaged tissue went on to repair itself. The new heart cells then began to beat in rhythm with the rest of the heart.
A group of researchers led by Melissa Little at the Murdoch Childrens Research Institute have produced a tiny kidney similar to that seen during foetal development. The kidney is a large and complex organ with many different types of cells, so it remains a challenge to grow an entire mature kidney the lab, but down the track, it may be possible to grow kidneys large enough to offer some sort of limited filtering function to patients. However, the ability to grow even these early stage kidney cells provides a valuable tool to study kidney development, carry out more directed research on specific mutations in kidney growth and to test new drugs.
All of this work feeds one of stem cell researchers’ biggest aspirations: being able to regrow or repair damaged human tissue, or even better, create entire brand new organs.
Not just growing new organs
Sometimes it’s not an entire organ that needs replacing. People suffering from some autoimmune diseases, where the patient’s immune system attacks its own body, may also benefit from developments in stem cell research, particularly where there is only a single type of cell affected by a disease.
Type 1 Diabetes
Type 1 diabetes occurs when a person’s immune system attacks the pancreas, destroying the cells that produce insulin. Without enough insulin, our body’s cells can’t absorb sugar properly and it builds up in the bloodstream, eventually damaging the kidneys, blood vessels and the retina.
A team led by Doug Melton have used embryonic stem cells to create insulin-producing cells that when transplanted into mice, responded to glucose by producing insulin. More research is needed to ensure this treatment is safe and effective in the long term and a viable option for people.
A further consideration is that transplantation of these cells in humans would have to be accompanied by treatments that curb the destructive action of the person’s immune system, so that the new cells aren’t damaged as well. An American company called ViaCyte is developing a system where stem cell precursors of insulin-producing beta cells are implanted into a patient within a protective ‘scaffold’. They then grow into mature beta cells capable of secreting insulin. The scaffolding protects the cells from the immune system’s antibodies that would destroy them and also prevents the cells diffusing into the body.
Scientists at Monash University and the University of Sydney have also used skin cells from patients with type 1 diabetes to develop iPS cells which they can use to study how the disease works and to test new drugs.
Multiple sclerosis
Multiple sclerosis (MS) is a disease where the body's immune system attacks its own myelin, which is a protective covering for the central nervous system.
This impacts upon the body’s nervous system to effectively transmit messages to the brain. A potential treatment involving haematopoietic stem cells for aggressive forms of the disease is currently in clinical trial stage in both the USA and Australia. It involves taking haematopoietic stem cells from the patient, then destroying their immune system with high-dose chemotherapy. The stem cells are then transplanted into the patient, with the hope that they will 'rebuild' a healthy immune system, without the damaging effects of the disease. Results from trials of this treatment and the small number of patients that have received the treatment have been mixed. While some patients do appear to benefit in the short term from the treatment, there is still no conclusive evidence that it will prove to be an effective long-term treatment.
Other research groups are trying to understand more about the role of stem cells in the brain and whether they can be reactivated to help combat the damage to nerves that occurs in multiple sclerosis.
Parkinson’s disease
Parkinson’s disease is a condition in which the dopamine-producing cells in a person’s brain degenerate and die, leaving the person unable to properly control their body’s movements. As there is only a small subset of nerves involved in this disease, it seems that stem cell therapy that aims to replenish those nerves could be a possible treatment. Scientists have been able to train both embryonic and iPS stem cells to become the specific type of brain cell that is affected by Parkinson’s disease. They have also been able to transplant these cells into the brains of mice, rats and monkeys, which improved some symptoms.
However, one of the issues with trying to treat Parkinson’s disease with stem cells is that several experiments have shown that after the stem cells are transplanted into a test animal’s brain, while some of them differentiate into the desired dopamine-producing neurons, many stem cells simply keep self-replicating, and form tumours. Clare Parish and Lachlan Thompson from the Florey Neuroscience Institutes and the University of Melbourne have developed a method where they can isolate the extra stem cells from the useful dopamine-producing cells in the lab, and discard the extra stem cells before they perform the transplant.
Work is continuing to ensure that this treatment doesn’t result in the growth of tumours, is effective in the long term and also to find ways to make the transplanted cells produce sufficient amounts of dopamine to restore function for Parkinson's patients. It is only through carefully conducted clinical trials that these challenges will be resolved.
Macular degeneration
A recent study found that stem cell therapy could be effective in improving the eyesight of people suffering from macular degeneration. Embryonic stem cells were turned into retinal pigment cells and injected behind the retinas of one of the study patients’ eyes. After monitoring their progress over three years, their eyesight had improved by several lines on a vision chart, and some of them were able to read a computer for the first time. Researchers were concerned about whether the stem cells might start proliferating without stopping, which could turn into tumours, but happily this did not eventuate in this study. The improvements found in the research are a good sign for future possible treatments for degenerative eyesight diseases but further refinement and testing, as well as long-term monitoring is required.
Still work to be done
Other stem cell treatments that make the headlines, such as treatments for spinal cord injury, offer some people hope when all other avenues seem to lead to dead ends. However, these treatments are not approved and have not yet gone through the rigorous clinical trial process to ensure that they are safe, let alone effective. Although there have been reports of miracle cures using stem cells, other outcomes of these experimental and unproven treatments have been less successful, and even tragic. We just don’t know enough yet about the long term consequences of stem cell treatments. Stem cell treatments offer huge promise, but it must be balanced with sensible caution.
Complications sometimes only show up down the track, as was the case with a woman who had stem cells from her nose implanted into her spine to try and treat paralysis. Not only did the treatment not work, but eight years later she needed surgery to remove a three centimetre growth of nasal tissue that had formed on her spine.
Disease in a dish
While growing new organs and fixing damaged spinal cords is definitely exciting and headline-making science, there is another less celebrated, but probably even more promising avenue for stem cell research: the ability to grow disease-affected cells. A sample of skin cells from a patient with particular diseases can be taken and reprogrammed to become stem cells. These iPS cells can then be turned into the diseased cells. Scientists can study the diseased cells to better understand both the disease’s progression and develop possible ways to treat it. It also provides an ideal guinea pig to test new drugs before trying them out on actual patients.
This is particularly useful for conditions that affect areas of the body that are difficult to take samples from, such as motor neurone disease, which affects the brain and spinal cord. This method also provides opportunities to study rare diseases that don’t always receive as much attention or funding as other more prominent conditions.
Researchers at the University of Queensland are investigating both Down syndrome and Alzheimer’s disease using iPS cells that were derived from patients; skin cells. Down syndrome causes brain changes that are similar to those that occur with Alzheimer’s disease. By developing the Down syndrome affected iPS cells into brain cells, the researchers can study the genetic characteristics of the diseases and attempt to identify pathways for treatments.
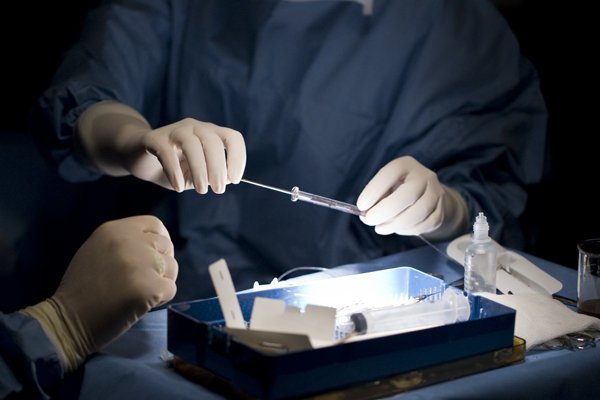
Researchers inject stem cells into retinas in an experiment to overcome blinding diseases. Image source: Bryan Jones / Flickr.
Ethical considerations
More than just where they come from
For some in the community, the announcement in the late 1990s that scientists were able to create stem cells from human embryos raised significant concern. They viewed the use of human embryos as destruction of human life, shrouding stem cell research in controversy. For others, the potential of medical research to help those suffering from incurable disease outweighed these concerns—particularly when stringent legislation was introduced in Australia that limited the use of human embryos to circumstances where the early stage embryos had been originally created for infertility treatment but were no longer required and would otherwise have been discarded.
Over the past decade, with strong public support for the use of stem cells in both research and treatment of disease, the debate has largely moved on. Controversy now lies in the land of the hope-peddling stem cell treatments that promise much but are less specific on what they can actually deliver. The hope that stem cell treatments can offer has spawned an industry where providers offer unproven and potentially unsafe experimental treatments … for those who are willing to pay.
In Australia, these sorts of treatments are generally offered by doctors operating outside of the clinical trial space. Overseas, the picture gets even more confused.
Stem cell tourism, where desperate patients and their carers travel overseas to countries to receive unproven treatments that are not offered in Australia for precisely that reason—they are unproven, and as such, could very well do more harm than good. With ‘do no harm’ being the central tenet of medical practice, doctors do not want to risk the health that patients do have on a risky and potentially threatening treatment.
Often the providers of these treatments make exaggerated claims about what they can achieve, without necessarily having any solid evidence, relying strongly on patient testimonials. But anecdotes are no substitute for impartial evidence obtained through rigorous research and a thorough clinical trial process. Playing on the perception that Australia is behind the times with respect to new stem cell treatments, or that medical doctors and researchers are in the pocket of Big Pharma who have agendas to restrict the treatments available to patients, these operators market a compelling solution—but at what cost? The reality is that we are at the very early stage of developing new treatments and progress needs to continue based on evidence rather than hype.
It’s clear that stem cells offer great hope for the future. That hope needs to be harnessed, and channelled into valuable research that will have the potential to benefit people suffering from a huge range of diseases and conditions with safe and effective treatments.